Documentation:FIB book/Airbag
Overview
Introduction
Airbags[1] are one of the most common safety systems in a car. But before the 1990s, it was uncommon for automobile manufacturers to equip airbags as standard equipment[2]. Since their introduction to the market, they continue to evolve in terms of their locations, shapes, and operation. And as a result of regulations and policies which mandate their inclusion in new vehicles, airbags have saved a significant number of lives. the National Highway Traffic Safety Administration (NHTSA) estimates that 50,457 lives have been saved by the activation of frontal airbags up to 2017 as recorded in the Fatality Analysis Reporting System (FARS), a nationwide US census[3].
Though given their contribution to vehicle safety during collisions, the airbags have become double-edged swords in certain scenarios as they themselves may cause injuries that wouldn’t otherwise be sustained by the occupants. For example, it is dangerous for vehicle occupants to lean against the door as side airbags may cause abdominal trauma[4][5]. There have also been incidents of airbag recalls. One of the notable ones is the recall of vehicles with Takata airbags as the airbags could be accidentally fired due to humidity and temperature changes[6]. The recall affects nearly 70 million vehicles in the US and the incident timeline spans nearly a decade, from 2014 to 2021[7]. The root cause of the safety risk is that the airbag's metal inflator is loaded with ammonium-nitrate-based propellant[7]. Changes in environmental factors, like temperatures and humidity, can cause airbags to be improperly inflated and also cause the metal airbag inflator housings to rupture during crashes[7]. In the US, 19 deaths and more than 400 injuries have been linked to inflator housings rupturing and sending metal shrapnel into vehicle occupants[7]. Moreover, airbags were primarily designed and positioned for adults rather than children, so there is an inherent risk of injury to children when exposed to a deploying airbag.
Because airbags can potentially cause significant harm to vehicle occupants despite their value in preventing adverse injury outcomes, we need to guide the airbag design/manufacturing industry and inform all consumers and vehicle manufacturers of the benefits and potential dangers of an airbag, whether the danger stems from inherent design flaws or inappropriate usage of them. This chapter of the book aims to do exactly that by discussing the various types and designs of airbags and ways to validate an airbag’s efficacy. This section will also showcase the shortcomings of existing airbag designs and finally, attempt to recommend future research directions in airbag design and testing.
Engineering Design Considerations of Frontal Airbags with a focus on deployment and inflation mechanics
Please see:
Types of Airbags
Locations
There are a variety of locations airbags can be mounted in a car to prevent different injuries. The standard locations for car airbags include:[1][8]
- Frontal Airbags: For the driver side this is typically built into the steering wheel, for the passenger side it is located in the dashboard near the glovebox. They prevent occupants from smashing forward into the dashboard or the steering wheel in a crash. They are designed to inflate in moderate to severe frontal crashes typically at speeds above 25 km/hr[1].
- Knee Airbags: These frontal airbags are mounted on both the driver and passenger side in the front seats near where the knees are located, in the area of the instrument panels. These airbags help keep an occupants legs in place and distribute impact forces to the legs in an attempt to reduce leg injuries.
- Roof Rail Airbags / Curtain Airbags: These side airbags are mounted along the roll cage of the car and deploy such that they cover along the top side of the length of the car on either side. They are at head height and prevent occupants from hitting their heads on the sides of the car.
- Side Impact Airbags / Side Torso Airbag: These airbags are at pelvic/thorax height and mounted into either the seats or the doors. These airbags typically deploy much faster than front airbags due to the occupant being closer to the door and thus there is a narrow time frame in which they can help.
- Combination Side Impact Airbags: Instead of both roof rail airbags and side impact airbags a car may have a combination side impact airbag that is mounted in the seat or door but inflates to cover both the pelvic/thorax area and the head area.
Components
Airbags typically consist of three main components; an impact sensor, an airbag cushion, and an inflation module.
The impact sensor is connected to the cars airbag control unit (ACU) which takes in the data from an impact and determines which airbags, if any, to deploy based on preprogramming.
The airbag cushion is what interfaces with the occupant to absorb the energy of the collision and reduce risk of injury. Newer airbags are specially shaped by the flexible fabric bag to better protect the occupant. The assembly must be fireproof and is typically made from nylon and polyesters which have high strength and elongation.
The inflation module can have a variety of chemical reactions but typically an igniter is used to start a reaction that rapidly produces primarily N2 nitrogen gas to inflate the airbag. Compressed gas canisters with a pyrotechnic operated valve are a common alternative. Airbags inflate at different rates depending on their location with side airbags inflating much faster than frontal airbags due to the limited space and thus time in which to deploy before the user comes into contact with what the airbag is meant to protect them from. The faster the airbag inflates the more risk there is to the occupant as it can apply forces to the occupant and injure them. Typically airbags inflate in approximately 0.04 seconds.
Effectiveness
Airbags aim to reduce the number of injuries following the maxims of restraint performance [9]. Airbags expand quickly in the case of a crash to maximize the time over which force is applied to the occupant thus reducing the peak force magnitude. rather than the force being applied all at once in the case of the user hitting something solid like the steering wheel it is instead drawn out over a distance taking power out of the system by the occupant deflating the airbag as they collide into it. Additionally airbags help to minimize the rotation or translation of an occupants joints and anatomical structure by restraining them. Finally an airbag can distribute what may have been a sharp impact into a impact distributed all over the body which can reduce the peak force to any one part of the body and distribute loads to more sturdy skeletal structures.
When evaluating an airbag’s efficacy, researchers first identify the high-risk body regions of interest, quantitatively determine the relevant injury criteria and rate the airbag depending on its ability to lower the injury metrics below the injury criteria. Often Head Impact Criteria (HIC) scores and Injury Severity Scores (ISS) are used, alongside ATD and cadaver testing as well as accident statistics and fatalities.
Frontal airbags reduce risk of death for drivers in frontal crashes by 29% and risk of death for front seat passenger 13+ years old by 32%[10].
Knee airbags have become increasingly common in vehicles. Their effectiveness is still widely debated with many studies finding minimal reduction in injury risk. A 2019 study by the IHSS found that in real-world crashes for seat belted occupants knee airbags only reduce overall injury risk by 0.5% with a statistically insignificant result[11]. While they likely do not reduce injury risk by much they also do not pose significant injury risk even in out of position scenarios[12]. It is suggested that knee airbags are more important for unbelted passengers as they serve a redundant purpose to lap belts which aim to keep the user in a seated position with the legs out in front of them, and this could be especially important for regions in the USA where seatbelts are not mandatory.
A car can come with just torso side airbags, combination side airbag, or both roof rail and side airbags. It has been found that torso only side airbags reduced car driver risk of death in driver-side crashes by 26% and for configurations with both torso and head side airbags the risk was reduced by 37%, and an even better reduction of risk of 52% for SUV style cars[13].
Novel airbag designs and applications
As airbag technologies matured over the years, automotive manufacturers have introduced innovative designs and implementations of airbag systems. Some are still offered in current vehicles after receiving strong market reception while others have morphed into other designs or informed manufacturers on other design aspects of airbags.[1] Below are a few note-worthy ones with their inception, reasoning behind their designs and their present-day status detailed. While not every novel airbag design below is mass produced and implemented in modern vehicle considerations, the intention is to use them as foundation to further research and develop future airbag technologies. By having a collection of innovative airbags, manufacturers can combine several solutions to mitigate disadvantages while synchronizing the benefits.
Inflatable Seat Belts
In 2011, Ford Motors Company introduced an inflatable seat belt as rear seat optional equipment for the 2011 Ford Explorer.[14] The availability of this option was later expanded to the Flex and Lincoln line up. Other Ford models, like the F150 adopted it through the 2010s However, the inflatable seat belt options were removed from some Ford vehicles of the 2021 year model in favor of implementing standard pretensioners and load limiters for the rear seat, in addition to the pre-existing pretensioners and load limiters on the front seats.[15] Interestingly, Mercedes-Benz continues to offer inflatable seat belts for the rear seats of the Mercedes-Benz S Class sedans.[16]
While Ford advertises the benefits of the inflatable seat belts are mainly in the reductions of head and chest accelerations during crashes, there is not a lot of literature that focuses on the biomechanical effects of inflatable seat belts or their efficacy in preventing injuries. However, Kevin et al. 2007, from the Ford Motor Company, performed sled testing on the efficacy of these seatbelts when paired with rear-facing-only child restraint systems in the rear seats[17]. The acceleration pulse corridor was in adherence with the Federal Motor Vehicle Safety Standards (FMVSS) 213 - Child Restraint Systems (CRS)[18]. They determined that while the lateral rotation of the ATDs and the CRS had more lateral rotation when constrained with the inflatable seat belts, the HIC36, the head injury criterion in 36ms time interval that measures the likelihood of head injury from an impact, in ATDs constrained with the inflatable seat belts were significantly lower than the ATDs constrained with standard seatbelts[17].
Far-side Airbags
The far-side airbag was introduced initially by General Motors in 2013, also known as the front-center airbag. The purpose is to prevent front passenger collision with each other during side-impact crashes and to maintain occupant position in far-side and rollover crashes. Following this design, Toyota designed a similar airbag, targeting rear-seat passengers.
In 2020, EuroNCAP, along with some car manufacturers, began to introduce and perform tests on the driver’s side of the car in a far-side impact[19]. This led to the implementation of a far-side airbag in several vehicles including the Chevrolet Traverse, GMC Acadia, Buick Enclave, Genesis G80. and Genesis GV80.
Panoramic Roof Airbags
In 2017, Hyundai Mobis introduced the panoramic roof curtain airbag, designed to prevent ejection of passengers through the sunroof in a rollover crash. The motivation behind such an innovative design was that with an increasing popularity in panoramic sunroofs in modern vehicles, the number of cases with ejection is expected to grow over the years. Based on NHTSA's 2000-2015 data, there are 260 identified occupants that have been ejected through the sunroof.
However, many challenges came with the implementation of this airbag, hindering its occurrence in the industry[20]. The panoramic sunroof airbag featured more technological difficulty and complexity compared to ordinary airbags due to space constraint when paired with the sunroof without interfering with headroom or visibility. Furthermore, the airbag must be deployed safely both when the sunroof screen is open or closed.
Seat Cushion Airbags
Beginning in 2014, the Toyota Yaris has been implementing a seat cushion airbag, designed to reduce forces on an occupant’s chest and abdomen by controlling movement of the body during a frontal crash. Both the airbag and its inflator module is positioned beneath the front passenger’s seat cushion, and is deployed upward and eventually swells up the front half of the seat cushion. This pushes the passenger’s legs up, preventing them from sliding forward and under the seat belt restraint.
Rear Seat Airbags
In 2019, Mercedes-Benz introduced an optional rear seat airbag in their S-Class sedans, designed to provide head protection for rear passengers in frontal crashes[21]. They are implemented in the back of the front seats and are deployed with less force than traditional airbags. Consequently, they are only partially inflated and are shaped differently in order to conform to smaller-sized occupants such as children.
Other Novel Airbags and Airbag Systems
Innovative design for airbags is still evolving in an attempt to maximize safety of passengers in motor vehicle collisions of all forms. While it is unlikely that there is one universal airbag that can protect a passenger in all scenarios, there are novel airbag designs that cover unique scenarios that can definitely happen to certain vehicles. Below are preliminary proof-of-concept designs that manufacturers hope to extend towards implementation in the future.
- flexible seating airbag systems
- external side airbag systems
- external hood airbags
Extending beyond airbags in cars, there are also innovative solution that focus on protecting passengers in small sized vehicles, such as motorcycles and bicycles.
- motorcycle airbags
- motorcyclist vest airbags
- bicycle helmet airbags
Airbag testing methodologies
For brain injuries, it is common to evaluate side airbags with criteria that take into account head resultant acceleration and magnitude of excursion. The most commonly used injury criterion is HIC[22]. The time interval used in calculating HIC is chosen to be 36ms or 15ms[23][24]. However, in 1999, the NHTSA adopted the 15ms evaluation interval of the HIC value and a maximum limit HIC15 value of 700 to be used in their FMVSS 208 regulation - Regulations for Vehicle Occupant Protections in Frontal Crashes[25]. Doing so made regulations for short-duration impact events more stringent[25]. For more information on calculating HIC, please refer to the Head section in this e-Text's Section 2: Injury Tolerance. It is common to correlate HIC with AIS codes as that relationship represents the correlation between head acceleration with injury severity.
It is also common for researchers to use the peak head resultant acceleration value directly, instead of taking into account the duration of the acceleration spike with HIC[26]. Usually, this is done with head-mounted accelerometers in PMHS or ATDs. The peak head resultant acceleration value without the side airbags is compared with the value with the side airbags installed and activated.
Another common method for measuring acceleration and accounting for the time durations of the kinematic events is to use image tracking solutions. In Umale’s side airbag tests with the THOR ATD, a 21-camera slow-motion video tracking system was implemented[26]. The system operated at 1000 frames per second to eliminate motion blur in accordance with SAE J211/2[27].
Similarly, another study that evaluated far-side airbags used a stereo photogrammetric system of 20 cameras that tracked external markers on the PMHS and WorldSID ATD at 1000Hz[28].
Detailing the common methodologies of airbag testing by showcasing some past studies as examples from the literature, the content of this section is categorized into 5 categories: Finite Element Analysis, Sled Testing, Crash Testing, Anthropometric Test Dummies (ATDs) and Postmortem Human Surrogates (PMHS). Within each category, the methods, results, and conclusions from one notable study that employed the specific airbag testing methodology are presented. Also, shortcomings and strengths of each study are discussed.
Finite Element Analysis
Because of the complexities of the models and the high costs of performing comprehensive sets of physical dummies or ATD laboratory tests, many turn to computational methods as they offer large freedom of flexibility. One notable study, done by Xingqiao et al. 2013, studied the parametric effects of a side curtain airbag deployment on ATDs' heads during side impact crashes and utilized finite element analysis (FEA)[29].
Instead of replicating vehicle-to-vehicle impacts, they simulated vehicle-to-moving deformable barrier (MDB) impacts, so they can modify a previously validated full-scale FE model, developed and validated by the National Crash Analysis Center (NCAC). Because they were using FEA, they could investigate a wide range of parametric effects which are the airbag-head boundary separation distance, the MDB velocity, the airbag trigger time, the initial airbag inlet temperature, and the number of particles to represent the amount of air inside the airbag. The considered parameters and their levels of magnitude are shown below.
In the past, it is observed that a decrease in factor A, the Airbag-head boundary separation distance increases the occupant injury risk [30]. Higher factor B (Moving Deformable Barrier velocity) means higher vehicle velocity during impact which leads to worse injury outcomes.
For the responses of interest, Xingqiao et al. 2013 decided to use the HIC 36 and the peak head acceleration values. As they needed to identify all five parameters' individual effects on the responses to determine their importance in airbag designs, they employed the design of experiments (DOE) methodology or the Taguchi method in designing their finite element model simulation[29][31]. Applying the DOE methodology helped them reduce the number of time-intensive FEA runs. Afterwards, they determined the relative influence and statistical significance of each parameter (shown in the above table) through the analysis of variance (ANOVA) technique.
They determined that aside from the MDB velocity, the initial airbag inlet temperature and the trigger time were the most influential factors[29]. All 5 factors' influences, in terms of their normalized significance, on the HIC36 and peak head acceleration are shown in the figure that follows[29].

Shown in the following figure, an increase in MDB velocity and trigger time, but not an increase in the initial airbag inlet temperature, significantly increased the HIC36 value[29].
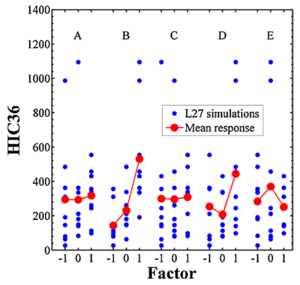
The airbag-head boundary separation distance, where your head is relative to the airbag, surprisingly did not have a significant influence on both the HIC36 and the peak head acceleration values[29]. This study demonstrates the effectiveness of side airbags in mitigating head injuries and the need for airbag manufacturers to focus on optimizing the airbag trigger time and initial airbag inlet temperature.
One critical benefit of finite element models is the degrees of freedom that they can offer. Without FEA, it would have been impractical and expensive to apply the DOE to actual sled testing. And if future researchers want to build off of the efforts of Xingqiao et al. 2013, they can do so more easily with FE models, rather than full-scale sled testing.
Sled Testing
One of the most important things in safety product verification is achieving high repeatability for all test repetitions. While repeatability can be achieved with computational methods, like the aforementioned finite element analysis, it is still a challenge in creating those models with realistic material properties. If the computational model, like an FEA model, uses the wrong material properties for a car interior, the output of the force and kinematic responses of the vehicle occupant model will not be realistic. This is commonly known as the Garbage In, Garage Out (GIGO) phenomenon in the computer science fields.
One way to combat GIGO and maintain the repeatability of tests is through the method of sled testing. In a sled test, the vehicle's interior is mounted in a reusable vehicle buck that is representative of the vehicle frame. The buck, with instrumented dummies inside, then is accelerated/decelerated to acceleration magnitudes representative of a crash. While the buck and the dummies experience acceleration patterns that are observed to cause injuries, the vehicle buck itself is not permanently deformed or damaged. This method, compared to crash testing, significantly reduces the costs of testing and increases repeatability as less deformation occurs.
One study that elucidated the efficacy of side airbags in reducing the risk of basilar skull fractures in a side impact is done by David et al. 2014[32]. In their study, they replicated side crashes at 15 and 20 mph crashes by inducing realistic acceleration pulses on vehicle bucks using sleds[32]. 5th percentile female Hybrid III dummies were positioned in a way that allowed their head form to repeatedly impact the B pillar or the combo side airbag during the sled testing[32]. They instrumented the head form with triaxial accelerometers at its center of gravity and integrated spinal transducers that measured the neck and lumbar force responses[32]. Sled testing reduces the chance of not being able to reuse the Hybrid III and its sensors after a testing trial since less destruction occurs in sled testing[32]. Below highspeed images from the study display how the Hybrid III was set up and its movement trajectory during a simulated side impact.
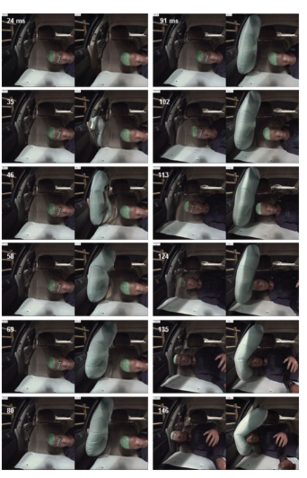
Despite the deployment of the side airbag, the risk of basilar skull fracture was not reduced[32]. Rather, the side airbags might have exacerbated the risks of injury despite David et al. 2014 observed the resultant head acceleration decreased from 93g without the airbag to 72g with the airbag[32]. The upper neck flexion moment was observed to be higher with the side airbag than without[32]. This increase in neck flexion moment is suspected due to the head being deflected forward by the airbag upon contact. In the highspeed videos, David et al. 2014 observed the head's movement[32]. Because of increased neck flexion moment, the upper neck compressive force was 207% of the Injury Assessment Reference Value (IARV) without the airbag and increased to 214% of IARV with the side combo airbag[32].
From their data, side combo airbags do not reduce the risk of basilar skull fracture, and further design work is required to prevent side airbags from deflecting the head and causing injury-inducing levels of neck flexion moments[32].
For future expansions:
Crash Testing
Anthropometric Test Dummies (ATDs)
Postmortem Human Surrogates (PMHS)
Shortcomings and Controversies of Airbag Design
Shortcomings
Studies have shown that side airbags (SABs) are effective in reducing fatalities and injury severity for near side impacts, especially designs which incorporate improved coverage. While there is a considerable amount of research done on the effectiveness of the different designs of side airbags, they are far from perfection. From a broad examination of research done into airbag design and performance, there are some clear shortcomings in either the method of evaluation or the design of airbags.
When considering the design of side airbags, they need to not exacerbate other injuries while reducing the risks of head injuries. More effort must be directed to solving the issue of occupants' head being deflected by the side airbags in other directions as redirection of travel induces neck flexion moment magnitudes several times larger than the IARVs[32]. Additionally, in the field of testing and validation, there lacks a standardized method of testing for SABs in general[33] which creates the possibility of inadequate amounts or quality of data being collected. Studies like Umale’s work on evaluating the effectiveness of airbags should not just take into account the peak head acceleration values, but also the time duration of the applied acceleration pulse[34]. This is an issue that could be addressed with a more standardized testing method in place.
Side Airbag Design Variations and Performance
While SABs are generally effective, certain designs have been shown to provide improved protection. SABs with improved head and thoracic protection have been shown to significantly reduce injury severity[35]. In a study done on the effects of SAB deployment in real-world crashes done by Loftis al[35], head and thoracic SAB performance was compared for designs with improved protection.
For head impacts both design with and without additional head protection performed similarly when evaluating for AIS 2+ injuries (45% of cases resulting in AIS2+ injuries vs 51.2%). However, when evaluating for AIS 4+ injuries, designs with additional head protection performed significantly better against designs without the added protection (22.2% vs 42.9%). This suggests that while even with the deployment of an SAB, head injuries of AIS 2+ severity are likely to occur, additional head protection can be beneficial for reducing the severity of head injuries
When evaluating for AIS 2+ injuries for thoracic impacts, the designs with added protection showed much better performance in comparison to the designs without (60% vs 75%). When evaluating for AIS 4+ injuries, the added protection design again outperformed the design without (38.9% vs 55.5%). This suggests that the additional protection designs are overall an improvement in reducing severity of injuries of all levels.
Limitations of Testing Methods
While airbag testing for frontal impacts typically use HIC, as mentioned earlier, currently for side airbags there are no standardized metrics to measure head injury for lateral impacts. Studies have used SID H3 ATDs to try and quantify injury risk for this loading condition[33]. Because of this, valuable data could be inadequately measured or completely missed. Additionally, it creates the possibility for discrepancies and variations in methods. Other implications from the testing methods include that it could prohibit or delay innovation in the design of airbags as automobile manufacturers do not have a standard to design around.
Potential Future Research Directions
Improved Head Injury Criterion for Lateral Impacts
Further SAB development is still being pursued. However, one barrier to current research is the lack of criteria to compare data to. Much of the data pertaining to lateral impacts is sourced from anecdotal data with no standardized testing. However, a significant barrier to implementing standardized testing procedures are the limitations on data collection. In the case of side impacts, one area of concern is the lack of injury criterion pertaining to head impacts. While compliance testing for the torso and pelvic regions have produced relevant side impact criterion such as the thoracic trauma index (TTI) and pelvic acceleration injury criteria, the primary injury criterion for the head, the head injury criterion (HIC), is based predominantly on data from frontal impacts[33]. Improvements in SAB technology would benefit from further research and understanding on lateral head injuries in addition to existing lateral thoracic and pelvic injuries. By creating a dedicated lateral head injury criterion based upon relevant data, current research can more accurately influence the future design and testing of novel SABs.
Out of Position Risks in Airbag Deployment
Out of position (OOP) scenarios occur when motor vehicle occupants are positioned in a manner that is outside of vehicle safety design. The wide range of potential positions are not well researched and are not tested under current standardized testing procedures. While comprehensive testing for all positions is unrealistic, the primary concern raised by preliminary research is that the use of airbags in some OOP scenarios may have a detrimental impact on occupant safety[36]. Elevated safety risks were found when evaluating injuries against neck injury criterion. Such risks warrant further research to provide guidance on the design of airbags to safeguard against OOP head injury and neck deflection.
Practice Problems
Problems
- A person was driving a 2016 Mercedes E-class wagon on Highway 1 westbound. Near his exit, he was distracted by his cell phone and did not see the constructions up ahead. When all the other drivers in front of him slowed down, he did not. This caused him to rear-end a 2022 Honda CR-V. The frontal airbags did not deploy due to a faulty automatic shut-off sensor. The chart below contains the resultant acceleration data of his head during the crash. How high was his HIC15? According to FMVSS 208, would this HIC be acceptable? Was an airbag potentially necessary in this case?
Answers
Using the time period from 40ms to 55ms, we calculate the HIC15 value with the following formula and t2 = 55ms and t1 = 40ms. Between t1 and t2, the acceleration integral is the largest.
The integral can be evaluated manually by dividing the irregular quadrilateral area into triangles and rectangles.
Then we can calculate the HIC15 value. The formula, with the variables replaced by the values, is entered into MATLAB and is shown below. This yields a HIC15 value of 4011.3. Given FMVSS 208 limits the HIC15 value below 700. this HIC15 value would not be acceptable. An airbag potentially could have lowered the HIC15 value by reducing the peak acceleration magnitude from 80Gs.
References
- ↑ 1.0 1.1 1.2 1.3 "Airbags". Airbags. The Insurance Institute for Highway Safety (IIHS). Retrieved Oct. 14th, 2022. Check date values in:
|access-date=
(help) - ↑ "Airbag". Airbag From Wikipedia, the free encyclopedia. Retrieved Oct. 14th, 2022. Check date values in:
|access-date=
(help) - ↑ "Traffic Safety Facts, Occupant Protection (2018 Data)" (PDF). National Highway Traffic Safety Administration, U.S. Department of Transportation. June, 2020. Retrieved Oct. 14th, 2022.
|first=
missing|last=
(help); Check date values in:|access-date=, |date=
(help) - ↑ Hallman, J. J., Brasel, K. J., Yoganandan, N., & Pintar, F. A. (2009). Splenic trauma as an adverse effect of torso-protecting side airbags: biomechanical and case evidence. Annals of advances in automotive medicine. Association for the Advancement of Automotive Medicine. Annual Scientific Conference, 53, 13–24.
- ↑ Hallman, J. J., Yoganandan, N., & Pintar, F. A. (2010). Biomechanical and injury response to posterolateral loading from torso side airbags. Stapp car crash journal, 54, 227–257. https://doi.org/10.4271/2010-22-0012
- ↑ "Takata Recall Spotlight". NHTSA. National Highway Traffic Safety Administration, U.S. Department of Transportation. Retrieved Oct. 14th, 2022. Check date values in:
|access-date=
(help) - ↑ 7.0 7.1 7.2 7.3 "Takata Airbag Recall: Everything You Need to Know". Consumer Reports. Sept. 21st, 2021.
|first=
missing|last=
(help); Check date values in:|date=
(help) - ↑ "Where are airbags located in my car". My Airbags. September 15, 2017. Retrieved December 04, 2022.
|first=
missing|last=
(help); Check date values in:|access-date=
(help) - ↑ Eppinger (2002) Ch 8, Occupant Restraint Systems, Nahum AM and Melvin JW (eds). Accidental Injury Biomechanics and Prevention. Springer Verlag, New York, NY
- ↑ Kahane, C. J. (2015, January). Lives saved by vehicle safety technologies and associated Federal Motor Vehicle Safety Standards, 1960 to 2012 – Passenger cars and LTVs – With reviews of 26 FMVSS and the effectiveness of their associated safety technologies in reducing fatalities, injuries, and crashes. (Report No. DOT HS 812 069). Washington, DC: National Highway Traffic Safety Administration.
- ↑ "IIHS researchers find little benefit from knee airbags". IIHS.org. August 7, 2019. Retrieved 2022-12-05.
- ↑ McMurry, Timothy & Forman, Jason & Shaw, Greg & Crandall, Jeff. (2019). Evaluating the influence of knee airbags on lower limb and whole-body injury. Traffic Injury Prevention. 10.1080/15389588.2019.1698737
- ↑ Anne T. McCartt & Sergey Y. Kyrychenko (2007) Efficacy of Side Airbags in Reducing Driver Deaths in Driver-Side Car and SUV Collisions, Traffic Injury Prevention, 8:2, 162-170, DOI: 10.1080/15389580601173875
- ↑ John, Neff (Nov. 5th, 2009). "Ford unveils industry's production inflatable seat belts". Autoblog. Retrieved Oct. 23rd, 2022. Check date values in:
|access-date=, |date=
(help) - ↑ Gustafson, Sven (Aug. 2nd, 2020). "Ford scraps optional inflatable rear seat belts from 2021 F-150". Autoblog. Retrieved Oct. 23rd, 2022. Check date values in:
|access-date=, |date=
(help) - ↑ Ewing, Steven (Sept. 6th, 2020). "2021 Mercedes-Benz S-Class safety tech: Rear-seat airbags and 48-volt architecture". Cnet. Retrieved Oct. 23rd, 2022. Check date values in:
|access-date=, |date=
(help) - ↑ 17.0 17.1 Pline, K., Board, D., Muralidharan, N., Sundararajan, S. et al., "An Assessment of Inflatable Seatbelt Interaction and Compatibility with Rear-Facing-Only Child Restraint Systems," SAE Int. J. Trans. Safety 5(2):2017, doi:10.4271/2017-01-1445.
- ↑ "LABORATORY TEST PROCEDURE FOR FMVSS 213 - Child Restraint Systems". Feb. 16, 2014. Retrieved Oct. 24th, 2022. Check date values in:
|access-date=, |date=
(help) - ↑ "Adult Occupant Protection Far-Side Impact". 2020. Retrieved 2022. Check date values in:
|access-date=
(help) - ↑ "Introducing the world's first panorama sunroof airbag system". 2017. Retrieved 2022. Check date values in:
|access-date=
(help) - ↑ "University of Extrication: Vehicle Airbags in the Seat Cushion". 2020. Retrieved 2022. Check date values in:
|access-date=
(help) - ↑ Qiu, J., Su, S., Duan, A., Feng, C., Xie, J., Li, K., & Yin, Z. (2020). Preliminary injury risk estimation for occupants involved in frontal crashes by combining computer simulations and real crashes. Science progress, 103(2), 36850420908750. https://doi.org/10.1177/0036850420908750
- ↑ Krzeminski DE, Fuss FK, Weizman Y, et al. Development of a pressure sensor platform for direct measurement of Head Injury Criterion (HIC).Proceed Eng 2015; 112:190–195.
- ↑ Jelen K, Fanta O, Billich R, et al. Whiplash injury and head injury criterion during deceleration.Trans Transp Sci 2011; 4(4): 217.
- ↑ 25.0 25.1 Eppinger, Rolf (November 1999). "Development of Improved Injury Criteria for the Assessment of Advanced Automotive Restraint Systems - II". National Highway Traffic Safety Administration.
- ↑ 26.0 26.1 Umale, S., Hauschild, H., Humm, J., Driesslein, K., & Yoganandan, N. (2019). Effectiveness of center-mounted airbag in far-side impacts based on THOR sled tests. Traffic injury prevention, 20(7), 726–731. https://doi.org/10.1080/15389588.2019.1650266
- ↑ "Instrumentation for Impact Test—Part 2: Photographic Instrumentation J211/2_202204". SAE International. April 14th, 2022. Retrieved Oct. 19th, 2022.
|first=
missing|last=
(help); Check date values in:|access-date=, |date=
(help) - ↑ Perez-Rapela, D., Donlon, J. P., Forman, J. L., Pipkorn, B., Shurtz, B. K., Markusic, C., & Crandall, J. R. (2021). Occupant Restraint in Far-Side Impacts: Cadaveric and WorldSID Responses to a Far-Side Airbag. Annals of biomedical engineering, 49(2), 802–811. https://doi.org/10.1007/s10439-020-02614-w
- ↑ 29.0 29.1 29.2 29.3 29.4 29.5 29.6 29.7 Deng, Xingqiao (March, 2013). "Finite element analysis of occupant head injuries: Parametric effects of the side curtain airbag deployment interaction with a dummy head in a side impact crash". Accident Analysis and Prevention (2013). 55: 232–241 – via Elsevier Science Direct. Check date values in:
|date=
(help) - ↑ Hallman, J. J., Yoganandan, N., & Pintar, F. A. (2010). Thoracic injury metrics with side air bag: stationary and dynamic occupants. Traffic injury prevention, 11(4), 433–442. https://doi.org/10.1080/15389581003753017
- ↑ Taguchi,G.,1987.System of Experimental Design, vol. IandII. UNIPUB, NewYork.
- ↑ 32.00 32.01 32.02 32.03 32.04 32.05 32.06 32.07 32.08 32.09 32.10 32.11 David C. Viano, Roger Burnett & Chantal S. Parenteau (2014) Influence ofa Combo Side Airbag on the Risk for Basilar Skull Fracture in a Far-Side Occupant, Traffic InjuryPrevention, 15:7, 726-733, DOI: 10.1080/15389588.2013.879124
- ↑ 33.0 33.1 33.2 Yoganandan, Narayan (2005). "Field Data on Head Injuries in Side Airbag Vehicles in Lateral Impact". Annu Proc Assoc Adv Automot Med. 49: 171–184.
- ↑ Umale, S., Hauschild, H., Humm, J., Driesslein, K., & Yoganandan, N. (2019). Effectiveness of center-mounted airbag in far-side impacts based on THOR sled tests. Traffic injury prevention, 20(7), 726–731. https://doi.org/10.1080/15389588.2019.1650266
- ↑ 35.0 35.1 Loftis, Kathryn (2011). "Investigating the Effects of Side Airbag Deployment in Real-World Crashes Using Crash Comparison Techniques". Ann Adv Automot Med. 55: 81–90.
- ↑ Potula, S.R.; Solanki, K.N.; Oglesby, D.L.; Tschopp, M.A.; Bhatia, M.A. "Investigating occupant safety through simulating the interaction between side curtain airbag deployment and an out-of-position occupant". Accident Analysis and Prevention. 49: 392–403.