Documentation:FIB book/Airbag/Considerations of Airbag Deployment on the Pediatric Population
Introduction

Airbags are a common safety feature in motor vehicles and are designed to protect vehicle occupants. Airbags can prevent serious and fatal injuries to the occupants in motor vehicle crashes; however, airbags were designed and positioned for adults, not children. This poses a serious threat to the pediatric population, specifically in children (ages 2-12 years), when in the passenger and rear seats of a motor vehicle.
According to the U.S. National Highway Traffic Safety Administration’s (NHTSA) Special Crash Investigations (SCI) database, 85 cases were under investigation involving children in 1998[2]. Out of these 85 cases, 24 sustained serious injuries, and 61 sustained fatalities. The passenger airbag was the source of injury in all these cases. In almost all these cases, the child was unrestrained or improperly restrained; however, airbags can cause injuries to children regardless of proper restraint[3]. This study demonstrates the importance of further research surrounding this topic and developing methods to mitigate serious injury. In the 1970s-1980s, studies found that the risk of injury was 26% lower for occupants seated in the rear seats of vehicles[4]. Due to this discovery, the NHTSA, NSC (National Safety Council), and various other organizations conducted campaigns in 1996 to move children to rear seats[4]. Fig. 1 demonstrates the decline of airbag deployment injuries in children after these safety measures were put in place. This was a very large step in advancing child safety in motor vehicles. Although this has made these injuries less common, they should continue to be evaluated as these injuries may affect how the child grows up and socially interacts with others. Common injuries due to airbag deployment in children include head and neck injuries, upper extremity injuries, and spine/ spinal cord injuries. This review aims to summarize the biomechanical knowledge of the airbag deployment injuries mentioned above in children. This review will also give rise to some unanswered questions that should be addressed to minimize and mitigate these injuries.
Head and Neck Injuries
From a child’s perspective, airbags are typically deployed towards their head and neck area, posing a severe risk of injury. Furthermore, a child’s position and improper use of restraints have contributed to increasing the severity level of a child’s injury in the event of an airbag deployment, particularly in the head and neck[3]. The first reported case of serious child injury where the cause was only airbag deployment and the child was properly restrained was in the mid 1990s[5]. The 3-year-old child was properly restrained in the front passenger seat in an approved forward-facing booster. The vehicle was in a low-speed right frontal collision, which caused only the passenger side airbag to deploy. Although the driver was uninjured, the child was decerebrate and apneic on scene. An MRI scan showed diffuse axonal injury (DAI) in several areas of the brain, but fortunately, the child began to recover after 1 month. The cause of injury was predicted to be due to the airbag directly hitting the child’s face when the body moved forward upon braking[5]. The impact caused rapid extension of the neck, which may have caused the DAI. It is clear that even in the case of a properly seated child, airbags have the potential to cause serious injuries to children. For an improperly seated or unrestrained child, there would be an even higher chance of serious injury, especially if the child is too close or thrown towards a deploying airbag. Other injuries that can occur as a result of airbag deployment on an unrestrained child include subdural hemorrhages, facial lacerations, and intraventricular bleeding[2][3]. As cases of airbag deployment-related injuries began to surface, researchers started to investigate methods for reducing injury risk to a child’s head and neck in the event of airbag deployment[6][7].
Surrogate (ATD) Testing

A study by Duma et al. investigated the dynamic response of the head and neck on an unrestrained 3-year-old Hybrid III ATD with side airbag loading[6]. Since the highest severity injury often comes from out-of-position (OOP) children, the study tested two irregular occupant positions that produced the most severe neck injury when simulated using multibody dynamics. Both positions represent a child sleeping with their head on the armrest, as shown in Fig. 2.
14 side airbag deployments were made on the 3-year-old ATD at four different airbag inflation levels[6]. To evaluate neck injury severity, a slightly modified, but more conservative, Nij definition was used to account for lateral bending in addition to tension and flexion of the neck.
Duma et al. have defined Nij > 1 as a 15% risk of AIS 3+ cervical spine injury, and Nij > 1.4 as a 30% risk of AIS 3+ cervical spine injury[6]. Out of all the tests, only position 1 at the highest level of inflation had a modified Nij of above 1 (1.09). The highest tension force (2317 N) was also observed in this case, which is slightly below the 2500 N injury threshold set by NHTSA in 1998 for a 3-year-old child[6]. From the test results, Duma et al. concluded that peak neck tension is correlated with airbag inflation properties, thus manufacturers should consider side airbags with variable inflator properties depending on the occupant’s mass[6].
Simulated Testing

In addition to conducting physical testing on ATDs, simulations have also been done to assess injury severity of children due to airbag deployment. A study by Sieveka et al. simulated the dynamic effects of an unrestrained 3-year-old child in various positions when impacted by a side airbag[7]. Several child positions were tested, as shown in Fig. 3. It is interesting to note that position 4 in this study is similar to position 2 of Duma et al.’s study (shown in Fig. 2), potentially indicating that this is a commonly perceived worst-case position for a child[6].
Comparing the simulated test results to NHTSA’s Protection Reference Values (PRVs), Sieveka et al.’s simulated shear and axial compression loads are lower than the recommended PRVs[7]. For shear loading, the highest simulated peak is near 400 N, as compared to the 1018 N recommendation. Likewise, for axial loading, the highest simulated peak is near 900 N, as compared to the 1313 N recommendation. Compared to Duma et al.’s study, the axial loading values are quite different[6]. This is potentially due to poor biofidelity of the 3-year-old HIII ATD’s neck and tendency to show a higher degree of injury than real-world data[8]. Different airbag properties and positioning of the child in the vehicle may have also contributed to the varied results. Duma et al. had noted high sensitivity to initial positioning on the child’s dynamic response in their study, as a change in 3cm reduced the resulting Nij by half[6].
Sieveka et al. simulated a peak acceleration of 180g in the worst case positions, which is much higher than the PRV value of 46g for 3-year-olds[7]. This value is in line with the values reported from Duma et al.’s study, where peak acceleration of the head ranged from 129g to 316g depending on the airbag inflator level[6]. From their work, Sieveka et al. concluded that when the head is close to the airbag’s point of deployment, high head accelerations may result, increasing the chances of acceleration-induced head injury[7]. Relating back to the first reported case of airbag-related serious child injury where the child was properly restrained, the high accelerations may have contributed to the child’s DAI[5]. This demonstrates the importance of research into injury due to high head accelerations, particularly in the case of airbag deploying towards children.
Discussion
Both studies discussed are examples of strong starts to encourage more researchers to investigate a child’s head and neck response in the event of airbag deployment, as this body region poses the greatest risk of serious injury upon impact. Duma et al.’s study provides useful data to inform the design of airbags with regards to inflation properties, while Sieveka et al.’s study provides useful information regarding child positioning relative to airbag point of deployment positioning[6][7]. However, there are several limitations of both studies. Duma et al.’s study was published in 1999, therefore the 3-year-old HIII is based on scaling the mid-size adult male HIII dummy and lacks biofidelity in the neck[6][8]. Sieveka et al.’s study was also published in 1999, so the validity of a multibody simulation of a 3-year-old is uncertain[7]. With limited cadaveric data for children, the scaled ATD and simulations cannot be directly validated. Additionally, no active neck muscle effects were taken into account for the simulations in Sieveka et al.’s study[7]. As a result, care must be taken when interpreting experimental data from these studies, as it may not be fully representative of the response of a child’s head and neck. Likewise, the injury threshold criteria published by NHTSA holds uncertainty because of the lack of child biomechanical data, which leads to uncertainty when comparing experimental data to injury thresholds. With improved biofidelity of child ATDs and new technologies, future studies can help better inform a more accurate injury threshold that airbag manufacturers can design towards, especially when making sure airbags do not pose a severe risk to children when deployed. Furthermore, in both Duma et al.’s and Sieveka et al.’s work, it is quite unlikely that children will be seated in the worst-case positions tested[6][7]. However, both studies further solidify the need for stricter regulations on appropriate car seats and positioning of children in vehicles to reduce the risk of head and neck injury during airbag deployment.
Spine and Spinal Cord Injuries
As mentioned in the Head and Neck Injuries section, the deployment of airbags impacts primarily the head and neck area, posing risk to the spine due to its close proximity. The cervical spine is closely associated with most fatal neck injuries since the spinal cord is at risk of injury upon damage of the cervical spinal column. Interest in pediatric spinal lesions was raised by Giguère et al. when they realized that reports omitted spine injuries as a cause of death[9].
Their study highlights cases where properly restrained children in the front seat sustained substantial damage to their spine due to airbag deployment[9]. In the most serious case, the 4-year-old child was wearing a lap belt and the motor vehicle was only travelling at 20 km/h; however, serious injuries were sustained[9]. The child was unresponsive, and further assessment of his injuries revealed unstable blood pressure, neck abrasions, and ecchymoses[9]. More importantly, the atlanto-occipital (AO) joint was found to be dislocated, and exposure of the spinal cord at the upper cervical spine levels caused a complete spinal cord transection at C1[9]. This resulted in serious spinal traumas such as quadriplegia, priapism due to spinal shock, and large hematoma in the cervical spine[9]. In light of so many possibilities of impactful traumas, researchers have invested more efforts in attempting to understand the biomechanics involving the spine and spinal cord when it comes to child occupants[10][11].
Surrogate (ATD and Animal) Testing


Child cadavers are relatively rare and due to the limited availability, there is not much data regarding children’s spine. To help bridge this gap, Prasad and Daniel conducted a study to observe responses to high accelerations from OOP frontal collisions with airbag deployment using child surrogates[10]. A dummy of a 3-year-old child and 12-15 week old piglets were subjected to sudden accelerations. Piglets were chosen to be an appropriate surrogate after judging the biomechanical factors, comparing anatomies and assessing the potential trauma sites[10]. In Fig. 4, the left schematic outlines the anatomy similarities between a human child and a piglet, while the right schematic shows the instrumentation for the dummy and the piglet, which both act as child surrogates.
Although the study was not entirely dedicated to spine response, several findings were related to the spine. For instance, there was a correlation between high spine acceleration, long duration of average acceleration, and high AIS injury levels for the brain and neck. 7 out of 15 piglets suffered from more severe AIS injury levels, as illustrated in Fig. 5[10].
Post-test autopsies revealed severe spinal cord injuries. These injuries include fatal three-fourths transection of the spinal cord at C1 level accompanied by subdural hemorrhage surrounding the spinal cord from the C1 to C4 level[10]. Since frequent cases of serious injury occur at specific sites like the C1 level of the spinal cord and the atlanto-occipital joint, it can be concluded that high tensile and bending stresses are applied at these regions of the spine when an airbag is deployed[10]. The superior-inferior (SI) acceleration of the piglet’s head was found to be a good representative value for accelerations that the atlanto-occipital joint is subject to[10]. From Table 1, it can be observed that severe to fatal neck injuries of AIS level 4-6 are associated with SI accelerations exceeding 100 g, potentially suggesting an injury threshold that airbag manufacturers can use to assess airbag safety for children[10].

Nightingale et al. also aimed to further understand the response of pediatric cervical spines to out-of-position airbag deployment[11]. However, they came to the same conclusion that the biomechanical data involving child spines are sparse and opted to conduct laboratory cadaver studies on adult cervical spines. A bending test and a tension test were performed. During the bending test, specimens were subjected to 30 repetitive cycles of pure flexion and extension while a six-axis load cell measured the loads applied at the base, as illustrated in Fig. 6 (left)[11]. For the tensile test, the test sample was mounted in a servo-hydraulic test frame, positioning it in an anatomically neutral position. The six-axis load cell was placed at the head’s centre of gravity to measure the tensile load applied along with displacement data. Fig. 6 (right) illustrates the setup for this test[11].
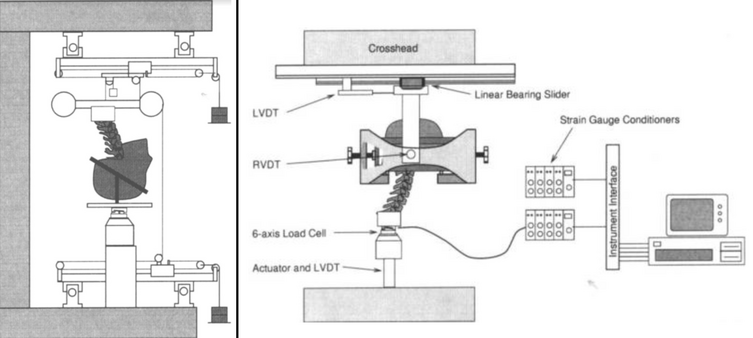
The results for the two tests are summarized in the tables below[11].

In both tests, results indicate that the upper cervical spine is two times stronger than the lower adult cervical spine. The average failure moment in flexion and extension for the upper cervical spine were 46.09 Nm and 44.70 Nm, while the lower cervical spine’s average failure moment in flexion and extension were 17.03 Nm and 18.09 Nm[11]. Results of the tensile test reveal an average load failure of 1996 N for the upper cervical spine[11]. This result provides further reason to realize the difference between children and adults when designing airbags, as the cervical spine remains most susceptible to serious traumas in children during airbag deployment, despite the higher strength demonstrated in adult cervical spines that airbags were designed for.
Discussion
Assimilating the information from the various studies, it is reasonable to conclude that injuries to the atlanto-occipital joint can cause severe spinal cord injuries. The deployment of high velocity airbags is highly likely to cause the dislocation of this craniovertebral junction in children. Gruyere et al. hypothesize that it is due to the head-to-torso ratio being much higher in children than in adults. This causes a greater momentum of the head movement after impact which in turn results in a cervical sprain or whiplash-like syndrome[9].
Prasad and Daniel’s study puts further emphasis on the atlanto-occipital joint being a vulnerable site by demonstrating the spine and spinal cord injuries sustained by the piglets and the dummy[10]. Although the anatomical similarity was justified enough to infer that similar kinematic and biomechanical responses are expected with an actual living child, surrogates still do not capture all parameters affecting kinematic responses and the number of samples was rather limited. The paper also attempted to set an injury criteria for the spine using the SI acceleration as a metric with the threshold being 100 g[10]. However, it is understandable to question the validity of this data since such a threshold was not demonstrated on living humans. With today’s technology, it is also possible to run simulations to confirm the results.
Nightingale et al. also expressed concern regarding the lack of biomechanical data due to spinal injuries, especially within the children demographic[11]. The paper also expresses similar views when it comes to differences in the pediatric cervical spine’s geometry and constitutive properties being a factor in cervical spine injuries due to airbag deployment[11]. The experiments revealed that upper cervical spine segments are stronger than their lower counterparts, but according to NHTSA data, 38% of adult motor vehicle accident cases sustained head or cervical spine injuries, while 98% of pediatric cases suffered from these traumas[11]. To further illustrate the differences between adults and children, 80% of cervical injuries occur in the upper cervical spine for individuals under the age of 16, while 84% of the adults’ cervical spine injuries occur between C3-C7[11]. This is potentially due to skeletal immaturity of young humans, which also explains why their spines are more prone to dislocations than fractures. The main limitation highlighted in this study remains the lack of testing on biofidelic specimens. Most conclusions drawn for the pediatric population are inferred or extrapolated from available surrogates which are possibly not accurate.
Upper Extremities Injuries
Upper extremity trauma is another common injury in children involving passenger airbags (PABs) with an AIS score of 2 or greater[12]. Arm injuries are one type of injury that has been found to be significantly different when comparing children that are properly restrained versus OOP[8]. As such, researchers have explored the effects of passenger airbags and child occupant positioning related to upper arm injuries[8][12].
Crash Case Studies

To further explore these injuries, a group from the Children’s Hospital of Philadelphia and the University of Pennsylvania analyzed real-life crash data extracted from insurance claims, telephone surveys, and crash investigations[12]. Specifically, the group focused on arm fractures in children exposed to PABs as they have been designed to attenuate head/neck injuries to decrease the mortality in adults, even though this is at the cost of increasing arm injuries. According to this study, most fractures occurred at the forearm, followed by the finger which can be seen in Fig. 7.
These fractures have been attributed to either direct impact on the airbag during deployment, or indirect impact on the vehicle's interior[12]. Before this study, no in-depth research has been done specifically on upper extremity fractures as this type of injury is rarely life-threatening. Despite this, the group found importance in this area of research due to how frequently upper extremity injuries occur and the potential developmental issues they may cause in children. Parameters such as types of injuries, injury severity, and crash severity were key in the statistical analysis on the crash data. Notably, delta V was calculated using crush measurements to get more quantitative data in terms of crash severity. The average delta V for the crash investigation data set was 25.4 ± 12.4 kph which can be identified as moderately severe in terms of vehicle accidents[12].
Based on this data, it was found that for children who were exposed to a PAB, 3.5% of them suffered an upper extremity fracture and 2.5 times more likely to sustain an upper extremity fracture, than those not exposed[12]. The researchers also established that lone upper extremity fractures were more common among female children compared to their male counterparts. This difference was attributed to the smaller cross-sectional area and bone density of the upper extremity bones seen in female children. Overall, this study outlined that the risk of injury for children is higher for those who are exposed to PABs compared to those who are not. In the case of vehicles with presumed depowered airbag capabilities, there was no significant difference found in occurrences of arm injuries compared to fully powered airbags, according to this set of data[12].

Sled Testing and Simulated Testing
The same research group with the addition of members from the Automotive Occupant Restraints Council also conducted a study on children in different restraint conditions[8]. Many studies have found a correlation between increased fatality risk for children who are OOP or improperly restrained[8]. To combat this risk, auto manufacturers have tried to suppress airbag inflation as a mitigation strategy[8]. This tactic was explored in this group’s study regarding upper extremity injuries and other injuries but found that further investigation would be needed to conclusively understand if this method is beneficial.


This study researched a similar set of real crash data and performed both sled tests and simulations to provide more evidence of the decision to depower airbags. To identify test parameters for both the sled testing and simulations, the group extrapolated from real-world crash investigations. The initial position of the three and six-year-old HIII ATD can be seen in Fig. 8 and 9. The baseline sled testing was used to verify the MADYMO models that were generated. New test conditions were then explored so that a variety of parameters could be explored in simulations. Finally, the second round of sled testing was done for key simulation test cases to verify the models further.
Similar to their previous study, it was found that there was almost double the chance of any injury when children were exposed to PABs which can be seen in Fig. 10[8][12]. The different body regions were looked at in more detail and found a significant difference between the risk of injury to the upper extremities when exposed to PABs compared to those who were not[8].
It was found in the simulation and sled testing that airbags, except when the child is properly restrained with a front chest and lap belt, can be beneficial to children when looking at specific indicators[8]. This trend was not seen in the real-life crash data. When looking at the depowered airbag data from the simulations and sled testing, it also seemed as though the risk of injury did not increase with the depowering of the airbag. These results were not significant enough to be seen as conclusive, making it a good area to continue research.
Discussion
Regarding the study on upper extremity fractures involving exposure to PABs, there were a few areas that were well-developed and others that could be improved[12]. As this study was mostly done on real-world data rather than biomechanical testing, it was insightful seeing delta V calculations done in order to characterize crash severity. This could not be done on every crash in their data set due to the lack of in-depth crash reports, but it was beneficial to give more concrete evidence when reporting the crash severity. In contrast, quantifying the injury severity was much more difficult as the estimations were solely based on phone call interviews with parents. Due to this, the AIS values proved to be somewhat arbitrary due to the lack of the parents' biomechanical knowledge. The claim that depowered PABs have no significant difference from fully powered PABs in terms of upper extremity injuries is also somewhat curious. This was based on estimates of car models' known features and whether or not they had depowering technology. Due to this, it would be best to do testing in the lab to capture results involving airbag inflation rates and pressures, similar to tests done by Duma et al. but with a focus on upper extremities[6].
The study researching OOP and improperly restrained children did good work further exploring the topic by using real-world crash data, ATD sled testing, and simulations[8]. It was great to see the time and care put into their experimentation process. The team tried to both gather evidence to support their initial test parameters through real data and verify both the input/output parameters going to their simulations with physical sled testing. It was also important that the researchers recognized that simulation testing of upper extremity injuries would be restricted due to the limits of the software as well as the lack of injury criteria for this part of the body. Based on this, it would have been nice to see a modified ATD being used with sensors placed on the upper extremities to get some experimental lab data if there is a lack of information to simulate these injuries with software. This would have been interesting since it was a significant indicator that was looked at in the real crash data analysis when assessing airbag depowering effects.
Future Research
Biofidelity
To make well-informed decisions when designing airbags while considering the pediatric population, manufacturers could benefit from further research that improves the biofidelity of ATDs representing children. The Hybrid III three-year-old dummy, used in the study performed by Duma et al., is a scaled version of the Hybrid III adult male[6]. The child ATD is based on scaling rather than biomechanical data because of the lack of data available on children. The validity of results is uncertain when scaling factors are used instead of biomechanical data. Seacrist et al. conducted a study to assess the biofidelity of the Hybrid III and Q series six-year-old dummies in low-speed, far-side oblique, and lateral impacts[13]. The study compared the biomechanical responses of both ATDs to the responses of child volunteers aged six to eight. Subjects were placed on a deceleration sled, which applied a crash pulse based on amusement park bumper car impacts. The study found that the ATDs overestimated the lateral bending and downward flexion of the head and neck. Seacrist et al also conducted a separate study to assess the biofidelity of the upper neck of the Hybrid III and Q series six and ten-year-old dummies in low-speed frontal crashes[14]. Pediatric ATD upper neck responses were compared to the biomechanical responses of child volunteers matched in size to the ATDs. To participate in the study, the child volunteers needed to match average mass and seated height of the ATDs within ±15%. Similar to the other study, participants were placed on an acceleration sled, which applied a crash pulse based on amusement park bumper car impacts. A 3D motion analysis system was used to track the movement of key points on the head and neck. The study found that the HIII 10, Q6, and Q10 ATDs underestimated peak axial load while the Q6 and Q10 ATDs overestimated bending moment. Both the axial load and bending moment are used to calculate neck injury criteria therefore impacting the certainty of calculations. Both studies were conducted under low-speed conditions and the results do not necessarily translate to high-speed impacts. Similarly, Menon et al. reported suboptimal biomechanical responses of the neck of the Hybrid III three-year-old dummy[8]. Future research should aim to ameliorate the biofidelity of pediatric ATDs by improving biomechanical data available on children, however, this may be difficult due to the lack of child cadavers.
Airbag Safety Improvements
Airbag safety improvements for children is another area of work which future researchers can look to improve. Graham et al have discussed strategies to reduce airbag risks to children[15]. To reduce risk, it is safer to have the child seated in the rear seat than seated in the front seat[15]. Child passenger safety laws vary by state and province, however, it is generally not advised to have children under the age of 12 seated in the front passenger seat. According to the CDC, children involved in car crashes in a state with booster seat laws were 20% less likely to die than children in states with no booster seat laws[16]. Despite the increased safety while seated in the rear seat, it has been found that one-third of children aged twelve and under use the front seat[15]. This suggests it may be worthwhile to investigate other strategies to reduce the adverse effects of airbag deployment on children/infants. One of the strategies discussed in this paper is to have vehicle manufacturers implement a child-detection feature in the front passenger seat. Some vehicles already use a weight sensor in the front passenger seat to detect whether someone is seated. If no weight is detected by the vehicle, the airbag will not deploy. The strategy is to have vehicle manufacturers modify this feature to suppress airbag deployment under a certain weight limit. With special permission, NHTSA has allowed car owners to install a switch to suppress airbag deployment for when a child is required to be seated in the front seat. However, there is controversy on whether this liberty should be granted given potential improper use by vehicle owners[15]. In order to understand whether suppressing airbag deployment provides greater benefits than deploying the airbag when a child sits in the front passenger seat, additional research is needed. Future researchers could also explore variable airbag inflation based on crash conditions such as dual stage airbags. These systems are currently implemented in many vehicles and inflate the airbag at two different powers depending on crash conditions. Additional research would be needed to determine the optimal inflation level for children. One of the most common injuries sustained by children from airbag deployment are upper extremity fractures[8]. Despite the high occurrence of upper extremity injuries, pediatric ATDs lack instrumentation in this region. Although it is not a high priority due to the low fatality risk of upper extremity fractures, future research can look to improve airbag safety in this region. Overall, future research should aim to address biofidelity issues of ATDs representative of children and to improve airbag safety for children and infants.
References
- ↑ A. Quiñones-Hinojosa et al, "Airbag Deployment and Improperly Restrained Children: A Lethal Combination," The Journal of Trauma, vol. 59, (3), pp. 729-733, 2005.
- ↑ 2.0 2.1 M. Kleinberger, N. Yoganandan and S. Kumaresan, "Review: Biomechanics of Child Occupant Protection," Crash Prevention and Injury Control, vol. 2, (1), pp. 63-73, 2000.
- ↑ 3.0 3.1 3.2 E. R. Grisoni et al, "Pediatric airbag injuries: The ohio experience," Journal of Pediatric Surgery, vol. 35, (2), pp. 160-163, 2000.
- ↑ 4.0 4.1 D. C. Viano and C. S. Parenteau, "Change in seating position of children in towaway crashes from 1989 to 2019," Accident Analysis and Prevention, vol. 159, pp. 106280-106280, 2021.
- ↑ 5.0 5.1 5.2 C. M. Hollands et al, "Severe head injury caused by airbag deployment," The Journal of Trauma, vol. 41, (5), pp. 920-922, 1996.
- ↑ 6.00 6.01 6.02 6.03 6.04 6.05 6.06 6.07 6.08 6.09 6.10 6.11 6.12 6.13 6.14 6.15 6.16 6.17 S. M. Duma et al, "Dynamic response of the Hybrid III three year old dummy head and neck during side air bag loading," Proceedings of the Institution of Mechanical Engineers. Part D, Journal of Automobile Engineering, vol. 213, (5), pp. 471-480, 1999.
- ↑ 7.0 7.1 7.2 7.3 7.4 7.5 7.6 7.7 7.8 7.9 E. Sieveka et al, “Three Year Old Child and Side Airbag Interaction Study using the CVS/ATB Multi-Body Simulation Program,” SAE Transactions, vol. 108, pp. 1416-1426, 1999.
- ↑ 8.00 8.01 8.02 8.03 8.04 8.05 8.06 8.07 8.08 8.09 8.10 8.11 8.12 8.13 8.14 8.15 Menon, R., et al. "Differences in air bag performance with children in various restraint configurations and vehicle types." 18th International Technical Conference on the Enhanced Safety of Vehicles, Nagoya, Japan, National Highway Traffic Safety Administration. 2003.
- ↑ 9.0 9.1 9.2 9.3 9.4 9.5 9.6 J. F. Giguère et al, "Airbags and children: A spectrum of C-spine injuries," Journal of Pediatric Surgery, vol. 33, (6), pp. 811-816, 1998
- ↑ 10.00 10.01 10.02 10.03 10.04 10.05 10.06 10.07 10.08 10.09 10.10 10.11 10.12 P. Prasad and R. P. Daniel, "A Biomechanical Analysis of Head, Neck, and Torso Injuries to Child Surrogates Due to Sudden Torso Acceleration," SAE Transactions, vol. 93, pp. 784-799, 1984.
- ↑ 11.00 11.01 11.02 11.03 11.04 11.05 11.06 11.07 11.08 11.09 11.10 11.11 11.12 Nightingale, Roger W. Roger W. et al, "Injury Mechanisms in the Pediatric Cervical Spine During Out-of-Position Airbag Deployments," Annual Proceedings - Association for the Advancement of Automotive Medicine, vol. 42, pp. 153-164, 1998
- ↑ 12.0 12.1 12.2 12.3 12.4 12.5 12.6 12.7 12.8 12.9 K. B. Arbogast et al, "Upper Extremity Fractures in Restrained Children Exposed to Passenger Airbags," SAE Transactions, vol. 112, pp. 540-547, 2003.
- ↑ T. Seacrist et al, "Evaluation of Pediatric ATD Biofidelity as Compared to Child Volunteers in Low-Speed Far-Side Oblique and Lateral Impacts," Traffic Injury Prevention, vol. 15, (S1), pp. S206-S214, 2014.
- ↑ T. Seacrist et al, "Evaluation of the Hybrid III and Q-Series Pediatric ATD Upper Neck Loads as Compared to Pediatric Volunteers in Low-Speed Frontal Crashes," Annals of Biomedical Engineering, vol. 41, (11), pp. 2381-2390, 2013.
- ↑ 15.0 15.1 15.2 15.3 J. D. Graham et al, "Reducing Risks to Children in Vehicles With Passenger Airbags," Pediatrics (Evanston), vol. 102, (1), pp. e3-e3, 1998.
- ↑ Centers for Disease Control and Prevention, "Child Passenger Safety: Get the Facts," cdc.gov. https://www.cdc.gov/transportationsafety/child_passenger_safety/cps-factsheet.html (accessed Dec. 3, 2021).