Course:CONS200/2023WT1/Next Gen Power? The Socioeconomic Benefits and Limitations of Geothermal Power in Iceland
Introduction
Iceland is at the forefront of sustainable energy thanks to it's vast geothermal resources. As of 2016, geothermal energy accounted for 65% of the total energy used in Iceland[1], and 97.4% of space heating in 2019[2]. Annually, 92 billion kWh of geothermal power is consumed across the world[1].Geothermal energy seems to be a promising alternative to burning coal and natural gas, with minimal ecological disruptions. Due to Iceland's location above a rift in the earth's crust, Iceland's utilization of Geothermal resources dates back centuries[3].
Ongoing advancements in the field and the expansion of geothermal power plants align with Iceland's vision of renewable energy generation and environmental stewardship. Geothermal power is a promising form of sustainable energy, however, consequences associated with operation need to be addressed in order to make geothermal energy truly sustainable. The primary negative consequences of geothermal energy in Iceland are side effects from depleting geothermal fluids, environmental pollution and habitat disturbance[4]. Harnessing geothermal power has hydrological and seismic consequences when geothermal fluids are extracted unsustainably[5][6]. Furthermore, geothermal power plants release hydrogen sulfide gas stored beneath the surface. Hydrogen sulfide is acutely toxic and chronic exposure has been linked to early deaths and increase rates of pulmonary diseases[7][8][9][10]. Lastly, Iceland's natural hot-springs are home to endemic species of thermophillic bacteria, mosses and lichens which are threatened by establishing geothermal power plants[11].
Geology
Epicentres of geothermal activity are often located at the edge of fault lines and rifts in the Earth’s crust[12]. Magma near the surface in these areas heats the bedrock, increasing in temperature as it gets deeper by 50-200º C per 100 metres. Groundwater is heated as well, and rises to the surface through fissures in the rock to form pools and hot springs[13]. Steam produced by geothermal sources can be tapped to spin turbines to generate energy and used as a source of central heating in cities[3].
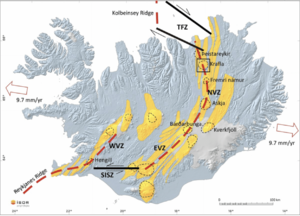
Iceland lies at the intersection of two oceanic rifts, the Mid-Atlantic Ridge and the Greenland-Iceland-Faeroe Ridge, as well as two chains of underwater volcanoes that follow tectonic plate boundaries[14]. It is one of the only places on Earth where submarine rifts rise above sea level, causing frequent volcanic activity.[15] The movement of the tectonic plates at their boundaries generates continuous volcanic activity, making Iceland a hotspot for volcanic eruptions and seismic events. The North American and Eurasian plates drift apart at the Mid-Atlantic Ridge, allowing magma to well up below the surface forming new crust and reshaping the terrain[16][14]. Constant plate movement creates fractures that spread across Iceland in narrow belts known as volcanic zones or volcanic fissure swarms[15].Volcanic fissure swarms can develop over time into active volcanoes surrounded by areas of high geothermic activity[14]. Reservoirs of super-heated steam are accessed by drilling into the Earth's crust, then transported into geothermal plants to heat water destined for district heating systems and to generate electricity [17]
While many of Iceland’s volcanic features have been dormant for 800 years, recent activity on the Reykjanes peninsula is a sign the island is waking up[18].
History of Geothermal Power in Iceland
The history of people in Iceland is tied to its volcanic activity. Even the name of the capital city Reykjavik comes from the old Norse word for smoke: an homage to the steam rising from the many hot springs[19]. Over the last century, advances in geothermal power plants have revolutionized mankind’s relationships with this resource. Geothermal power was first introduced to supply central heating in the 1930s. Before the 1930s, Iceland was dependent on imported coal to meet its energy needs[20]. After heating a trial section of 50 houses proved to be a success, the city of Reykjavik began a complete overhaul of the local infrastructure in order to shift to geothermal heating. The transition began in 1939 and was completed in 1943, construction having been delayed by the outbreak of WWII. The switch to geothermal heating occurred during a period of growth, when increased urban construction, and the steep price of coal were strong motivators for a new kind of energy[20]. The shift towards geothermal heating not only reshaped urban landscapes, but established Iceland as a pioneer of geothermal energy use and sustainable energy solutions [21].
Current Usage
Heat and Electricity Generation
Geothermal energy more than exceeds Iceland's needs for heat[22]. Fresh water is heated by contact with pipes containing geothermal fluids, then transported to cities where it flows through space heaters and underneath sidewalks and roads to melt snow or ice. Most geothermal power plants are equipped with infrastructure capable of heating water as well as generating electricity[23].
Geothermal power generation in Iceland is accomplished by harnessing heat energy in water super-heated by the Earth's core. Water deep beneath the surface is heated by nearby volcanic activity causing it to boil to the surface as steam exiting a natural geyser. To harness the energy supplied by the Earth's core, a borehole is drilled into the Earth's crust to access steam that would otherwise be destined for natural geysers, or to pump fluid into artificial reservoirs. Steam is channeled through pipes, into turbines, then re-condenses in a containment chamber as water. The turbines convert mechanical energy supplied by the movement of steam into rotational energy around a crankshaft inside the turbine. Finally, the crankshaft is attached to a generator, which converts the rotational energy into electricity[24].
Modern Developments
Geothermal power production is historically an inefficient process. Heat is used to spin turbines and is lost to the environment[25]. Modern innovations in power plant design center around increasing efficiency while mitigating ecological ramifications. Computer models of power plant thermodynamics allow researchers to isolate and adjust parameters to test for desired outcomes.
In many cases, operations of geothermal power plants are manually controlled, and the focus is on operation safety and production of required power, not improving efficiency. Implementing a proportional-integral-derivative (PID) could maximize efficiency without sacrificing worker safety. The PID is responsible for controlling power output by scaling the measured speed up or down to match the desired speed and is standard in many industries. A thermodynamic computer model generated in 2020 found that automation by a PID controller would increase the exergy efficiency by 25% and the power generation by 23%. The cost of this installation however could add to the already high price of geothermal energy infrastructure[26].
Consequences of Geothermal Power
Effects of Fluid Withdrawal
Drilling into the Earth and removing water from natural aquifers has a wide variety of hydrological and seismic consequences. Withdrawal of geothermal fluids can result in natural hot-springs disappearing or becoming fumaroles, a vent where only geothermal gases are released [5]. Depleting geothermal fluids reduces the average groundwater level, potentially causing aquifers below the surface to mix together. If an aquifer supplying clean drinking water were to become contaminated with toxic geothermal fluids, the long term consequences for water quality would be considerable[27]. Lastly, natural geological formations may change or disappear, having downstream effects for endemic biodiversity[5].
Fortunately, re-injection of spent geothermal fluids is a common practice that address these concerns effectively [28]. However, the process of re-injection itself has been linked to induced seismic activity in geothermally active areas. Because spent geothermal fluids are significantly colder than fresh geothermal fluids, large temperature differences cause rapid expansion and contraction of the surrounding mantle, encouraging seismic activity[6][29].
Habitat Disturbance
Iceland's natural hot-springs are unique ecosystems full of heat energy available to be converted into electricity at a geothermal power plant. Infrastructure required for the creation and operation of geothermal plants disrupts the ecosystem through habitat fragmentation and landscape change[11].Roads built in order to begin the construction process disrupt the delicate ecosystems surrounding hot-springs, or the natural tundra[11]. Long term ecological consequences after the establishment of a geothermal plant include habitat fragmentation and landscape change.
Iceland's hot-springs are home to novel examples biodiversity such as thermophillic bacteria and other potentially unique microorganisms, however these do not seem to be directly affected by drilling operations[27]. Stability of this ecosystem is threatened when large amounts of heat and water are removed as geothermal fluids[5]. Unsustainable extraction of geothermal fluids threatens thermophillic bacteria reliant on heat, as well as unique species of moss and plants directly affected by changes in hot-springs[27]. Once geothermal plants have been established, operation contributes much less to habitat disturbance than creating infrastructure for construction[27]. However, geothermal pollution presents health risks due to hydrogen sulfide gas and heavy metals present in geothermal fluids. Recent studies have found increased concentrations of trace elements such as arsenic and boron in subarctic soils and mosses near geothermal power plants [30][31]. These findings align with previous research linking increased concentrations of trace element in the livers of small mammals near geothermal plants [32].
Hydrogen Sulfide Pollution
Geothermal fluid contains hydrogen sulfide and carbon dioxide gas which is taken from below the surface and released into the air[7]. Hydrogen sulfide is a naturally occurring compound, even being used endogenously in humans as a signaling molecule[33]. Carbon dioxide is infamous for its role in climate change, however, hydrogen sulfide concentrations in the air near geothermal power plants has been linked with early deaths and associated with increased rates of pulmonary disorders[8][9][10]. However, results are not conclusive. Other evidence has found no association with pulmonary disorders, and even a slight reduction in pulmonary diseases suggesting hydrogen sulfide may be beneficial for lung health[34]. Regardless, hydrogen sulfide exposure will need to be studied further due to existing evidence for negative consequences.
Economic Impacts
Background
As a sustainable energy production technology, geothermal systems are a promising alternative to fossil based fuels and demonstrate the potential for economic growth[35]. For example, previously one of the poorest countries in Europe in the 20th century, Iceland was heavily dependent on imported coal for their energy sector [22]. However, Iceland became energy independent by transitioning to sources of domestically produced renewable energy. in 2014, renewable energy accounted for 85 percent of Iceland's energy use, 66 percent of which is attributable to geothermal energy [22]. Furthermore, a statistically significant relationship between renewable energy utilization and increase in green GDP has been found, which contributes to an increase in overall GDP[36].
Transitioning from Fossil Fuels to Geothermal Energy
In the short term,geothermal power plants are less profitable relative to other sources of renewable energy, due to higher initial investment costs and a longer return on investment[35]. Combined this presents a high investment risk which can slow down, or prevent the construction of a plant completely[35]. Additionally, limited knowledge of how viable a geothermal reservoir is prior to construction creates further risk[35]. Furthermore, investment costs are relative to the known viability of a site, with costs decreasing as information about a site's viability increases[35].
Surface surveys, subsurface explorations and experiments determine whether a geothermal reservoir is viable[35]. On average, the process of determining if a geothermal field is viable takes around two to three years, with an additional three to five years required for construction of the geothermal plant[35]. The two main obstacles that impede geothermal development are a high initial investment, and the uncertainty the early steps of development[35]. Furthermore, the performance of the power plants vary as the ambient temperature constantly changes, and the performance of the plants decrease over time bringing profits down with it[35].
To address these problems, hybrid power plants have come to fruition, which combine additional renewable energy sources to diversify energy production[35]. Combining geothermal with wind or solar energy helps to reduce operating and investment costs, shortening the payback period[35]. In one case, the LCOE (levelized cost of energy) of hybrid geothermal plant was reduced from $225/MwH, to $165/MwH with solar energy accounting for 48% of energy production[37].
Additionally, some countries are implementing a program of loan guarantee, where if the borrower does not repay the loan on time, the policy guarantees the third party guarantor to pay the loan back[35]. For example, in Germany the “Risk of Non-Discovery of Deep Geothermal Energy” guarantees a maximum of 100 percent of the loan money being paid[38]. Additionally, drilling operation insurances, loan support mechanisms and grants are available to reduce the investment risk involved with developing a geothermal energy plant[35].
Transitioning to geothermal energy increases domestic energy production and usage, which can lead Iceland’s usage of fossil fuels to decrease. This is important as economic growth is positively influenced by the production of renewable energy[39]. In turn, geothermal energy offers an independence from oil and gas that increases a country’s energy security while increasing employment opportunities[35]. In turn, Iceland is able to increase job opportunities and reduce reliance on imported fossil fuels, creating room for economic growth. For example, using geothermal energy can save the equivalent of 26.2 million tons of oil every year[40], which on its own, alleviates a large overhead cost for energy production.
Levelized Cost of Energy
An important concept to consider relative to energy production is the Levelized Cost of Energy (LCOE), which accounts for initial investment, operation costs, power generation rate, power plant life, cost reduction rate and availability of facilities[41]. The LCOE is measured as costs relative electricity production over a plant's lifetime. Alternatively, LCOE is the price to generate 1 kilowatt per hour ($/kWh), where the lower costs of electricity generation results in a cheaper kilowatt hour and lower LCOE. Relative to LCOE values, it has been found that geothermal energy is more efficient than some other renewable energy sources, and in South America, has the lowest $/kWh compared to all renewable energy sources across 9 global regions[35].
Reliability of Geothermal Energy
Geothermal energy is a relatively reliable source of renewable energy once established. Relying on geothermal reduces economic variability from changing oil prices[35],[42]. Additionally, unlike other renewable energy sources such as wind or solar energy, it may not be affected by climate change[35]. The higher reliability of geothermal systems has strengthened Iceland's economy, and creates broad support for national energy security [42].
Geothermal Energy and its Effect on Tourism
However, although there is potential for significant economic benefits by utilizing geothermal systems, these benefits are contrasted by potential negative impacts on other areas of the economy. Geothermal projects can affect local hunting grounds, pastures, historical parks, national parks, geysers or hot springs, which in turn can affect tourism [27]. This is important as one of Iceland’s largest exports is tourism thanks to the natural landscape [43]. It has been suggested that renewable energy infrastructure, including geothermal systems, reduces the attractiveness of nature-based tourism destinations [43]. However, despite this idea, tourism in Iceland has grown exponentially, increasing an average of 13 percent per year between 1999 and 2019, and has seen annual growth of 22 percent since 2010[43]. Interestingly, some tourists also name geothermal areas as a reason for travel , which in turn can potentially increase tourism further [44]. Therefore, geothermal energy may disturb the landscape and sublimity of nature, however tourism seems to be unaffected within Iceland, and has contributed to further economic growth.
Mitigation Strategies
Based on the following information three main problems has been addressed with potential solutions that highlight these factors.
Side Effects of Depleting Geothermal Fluid
The excessive extraction of geothermal fluid can lead to a decline in reservoir pressure, resulting in reduced energy production and potential long term damage to the geothermal resource[45]. A potential solution is the implementation of sustainable reservoir management practices, such as the re-injection of treated or cooled fluid back into the reservoir to maintain pressure levels [45]. This process, known as re-injection or injection of spent geothermal fluids, helps replenish the reservoir and sustains long term energy production. Moreover, achieving optimal and efficient management of geothermal resources necessitates a thorough comprehension of the present industry landscape. The complexity arises in identifying the most effective re-injection strategies, given that each geothermal field possesses distinct geological settings and reservoir characteristics[45]. High re-injection rates can be a factor contributing to ground inflation or uplifting, causing an increase pore pressure or fault slip leading to deformation of the surface. To avoid this problem, deploying strategies of redistribution of injection amounts over greater surface area can help mitigate this situation[46].
Habitat Disturbance Through Landscape Change
The development of geothermal energy infrastructure, such as clearing sites, drilling wells and constructing power plants, can cause habitat disturbance and alter the landscape, impacting local ecosystems and wildlife[47]. Drilling and seismic surveys may result in erosion, runoff and noise which disturb surrounding habitat, as well as native vegetative species[48]. While the environmental effects of geothermal power generation and direct utilization are generally deemed minor or manageable, the implementation of suitable mitigation measures can further minimize these impacts. A potential solution brought to light addressed incorporating the implementation of a comprehensive environmental impact assessments before geothermal projects commence [48]. This includes identifying sensitive areas and implementing mitigation measures such as re-vegetation, habitat restoration, and land-use planning to minimize the impact on local ecosystems. Additionally, involving local communities and environmental organizations in the planning process can help address concerns and find solutions that balance energy needs with environmental conservation[49].
Heat/Chemical Pollution from Geothermal Waste Water
The discharge of geothermal wastewater containing heat and potentially harmful chemicals into surface water bodies can lead to thermal pollution, adversely affecting aquatic ecosystems and lead to undesirable vegetation growth[48]. This increased water temperature has additionally lead to a reduction in the concentration of dissolved oxygen in water, posing a potential thread to aquatic organisms[48]. A potential solution would employ effective wastewater treatment technologies to remove harmful chemicals and reduce the temperature of geothermal wastewater before discharge. The implementation of advanced treatment processes, such as evaporation ponds, biological treatment, and chemical precipitation, can help ensure that the discharge water meets environmental quality standards [50]. In addition, to minimize the environmental impact, the consideration of utilizing treated wastewater can be beneficial for purposes such as agriculture irrigation, as well as livestock and wildlife watering [51].
Conclusion
Iceland stands as a pioneer in sustainable energy use, capitalizing on its abundant geothermal resources shaped by a unique geological setting above a rift in the earth's crust. Geothermal energy, constituting a significant proportion of Iceland's energy mix, aligns with the country's vision of environmental stewardship. With lower greenhouse gas emissions compared to traditional fossil fuels, geothermal energy offers a promising alternative. However, as with any energy source, it comes with its set of challenges. Consequences of geothermal power, such as fluid withdrawal effects, habitat disturbance, and hydrogen sulfide pollution pose challenges that demand careful consideration.
Mitigation strategies, including sustainable reservoir management, comprehensive environmental impact assessments, and advanced wastewater treatment, are vital in addressing the ecological concerns associated with geothermal projects. These strategies emphasize the importance of considering the unique geological and environmental characteristics of each geothermal field.
Economically, Iceland's transition from fossil fuels to geothermal energy has proven beneficial, contributing to increased energy security and economic growth. Yet, challenges in terms of initial investment costs and resource viability uncertainties underscore the need for innovative approaches, such as hybrid power plants and financial support mechanisms.
Looking ahead, Iceland's geothermal energy sector holds promise for sustainable energy production and continued economic growth. Strategic collaboration among policymakers, researchers, and industry stakeholders, coupled with ongoing investments in research and development, will be crucial in ensuring the long-term viability and positive impact of geothermal energy in Iceland. As the nation navigates the delicate balance between progress and environmental sustainability, careful planning and innovation will be key to securing a bright and sustainable future powered by geothermal energy.
References
- ↑ 1.0 1.1 "Geothermal Explained - Use of Geothermal Energy". U.S Energy Information Administration. April 2023.
- ↑ Hjaltason, Elías Sigurður; Guðmundsdóttir, María; Haukdal, Jón Ásgeir; Jón, Ragnar Guðmundsson (2021). Energy Statistics 2020 (PDF). Iceland: National Energy Authority. pp. page 3, page 6.CS1 maint: extra text (link)
- ↑ 3.0 3.1 Barnea, Joseph (January 1972). "Geothermal Power". Scientific American. Vol. 226: pp. 70-77 – via JSTOR.CS1 maint: extra text (link)
- ↑ Kristmannsdóttir, H., & Ármannsson, H. (2003). Environmental aspects of geothermal energy utilization. Selected Papers from the European Geothermal Conference 2003, 32(4), 451–461. https://doi.org/10.1016/S0375-6505(03)00052-X
- ↑ 5.0 5.1 5.2 5.3 Glover, R. B., Hunt, T. M., & Severne, C. M. (2000). Impacts of development on a natural thermal feature and their mitigation—Ohaaki Pool, New Zealand. Geothermics, 29(4), 509–523. https://doi.org/10.1016/S0375-6505(00)00019-5
- ↑ 6.0 6.1 Ji, Y., Hofmann, H., Duan, K., & Zang, A. (2022). Laboratory experiments on fault behavior towards better understanding of injection-induced seismicity in geoenergy systems. Earth-Science Reviews, 226, 103916. https://doi.org/10.1016/j.earscirev.2021.103916
- ↑ 7.0 7.1 Olafsdottir, S., & Gardarsson, S. M. (2013). Impacts of meteorological factors on hydrogen sulfide concentration downwind of geothermal power plants. Atmospheric Environment, 77, 185–192. https://doi.org/10.1016/j.atmosenv.2013.04.077
- ↑ 8.0 8.1 Carlsen, H. K., Zoëga, H., Valdimarsdóttir, U., Gíslason, T., & Hrafnkelsson, B. (2012). Hydrogen sulfide and particle matter levels associated with increased dispensing of anti-asthma drugs in Iceland’s capital. Environmental Research, 113, 33–39. https://doi.org/10.1016/j.envres.2011.10.010
- ↑ 9.0 9.1 Ragnhildur Gudrun Finnbjornsdottir, Anna Oudin, Bjarki Thor Elvarsson, Thorarinn Gislason, & Vilhjalmur Rafnsson. (2015). Hydrogen sulfide and traffic-related air pollutants in association with increased mortality: A case-crossover study in Reykjavik, Iceland. BMJ Open, 5(4), e007272. https://doi.org/10.1136/bmjopen-2014-007272
- ↑ 10.0 10.1 Bates, M. N., Garrett, N., Crane, J., & Balmes, J. R. (2013). Associations of ambient hydrogen sulfide exposure with self-reported asthma and asthma symptoms. Environmental Research, 122, 81–87. https://doi.org/10.1016/j.envres.2013.02.002
- ↑ 11.0 11.1 11.2 Albertsson, A., Blondal, A., Barkarson, B. H., & Thors, S. G. (2010). Environmental Impact Assessment of Geothermal Projects in Iceland. https://api.semanticscholar.org/CorpusID:112922590
- ↑ "Geothermal Explained - Where Geothermal Energy is Found". U.S Energy Information Administration. February 2022.
- ↑ Thordarson, Thor (2012). "Outline of Geology of Iceland". The Wayback Machine. Archived (PDF) from the original on March 27 2016
|archive-url=
requires|url=
(help). Check date values in:|access-date=, |archive-date=
(help); Missing or empty|url=
(help);|access-date=
requires|url=
(help) - ↑ 14.0 14.1 14.2 Tobias, Weisenberger (2010). "Introduction to the Geology of Iceland". Iceland.
- ↑ 15.0 15.1 Chapman, Thordarson (2012). "Outline of the Geology of Iceland" (PDF). Web Archive.
- ↑ Bergerat; Sæmundsson; Fourel; Angelier (14 July 2017). "Structure and activity of the geothermal field of Hvalfjörður (Iceland) from brittle tectonic, geothermal and paleostress analysis". Bulletin de la Société Géologique de France: 451–465 – via GeoScience World.
- ↑ Arnórsson, Stefán (20 January 2000). "Geothermal systems in Iceland: Structure and conceptual models—I. High-temperature areas". Geothermics: 561–602 – via Elsevier.
- ↑ Andrews, Robin (March 22 2021). "Eruption in Iceland may mark the start of decades of volcanic activity". National Geographic. Retrieved 21 November 2023. Check date values in:
|date=
(help) - ↑ Sveinbjörnsdóttir, Emilía (March 2002). "Is there a place name in the capital area or a bay or an inlet called Reykjavík?". Vísindavefurinn – via The Source Web.
- ↑ 20.0 20.1 Melsted, Odinn (2019). "Who Generates Demand for Sustainable Energy Transitions? Geothermal Heating in Reykjavík". RCC Perspective: pp. 31-38 – via JSTOR.CS1 maint: extra text (link)
- ↑ Spittler; Davidsdottir; Shafiei; Leaver; Asgeirsson; Stefansson (28 February 2002). "The role of geothermal resources in sustainable power system planning in Iceland". Renewable Energy: 1081–1090 – via Elsevier.
- ↑ 22.0 22.1 22.2 "Geothermal". Government of Iceland. n.d. Check date values in:
|date=
(help) - ↑ "Hellisheidi Geothermal Power Plant, Hengill, Iceland". Power Technology. 13th September 2023. Retrieved December 10th 2023. Check date values in:
|access-date=, |date=
(help) - ↑ "Electricity Generation". Office of Energy Efficiency and Renewable Energy. December 10th 2023. Retrieved December 10th 2023. Check date values in:
|access-date=, |date=
(help) - ↑ Zarrouk, S. J., & Moon, H. (2014). Efficiency of geothermal power plants: A worldwide review. Geothermics, 51, 142–153. https://doi.org/10.1016/j.geothermics.2013.11.001
- ↑ Çetin; Özkaraca; Keçebaş (4 December 2020). "Development of PID based control strategy in maximum exergy efficiency of a geothermal power plant". Renewable and Sustainable Energy Reviews – via Science Direct.
- ↑ 27.0 27.1 27.2 27.3 27.4 Kristmannsdóttir, Hrefna; Ármannsson, Halldór (August 2003). "Environmental aspects of geothermal energy utilization". Geothermics. 32: pp. 451-461 – via Science Direct.CS1 maint: extra text (link)
- ↑ Brandsdóttir, B., Franzson, H., Einarsson, P., Árnason, K., & Kristmannsdóttir, H. (1993). Seismic monitoring during an injection experiment in the Svartsengi geothermal field, Iceland. Jökull, 51, 43–52. https://doi.org/10.33799/jokull2002.51.043
- ↑ Cao, W., Durucan, S., Shi, J.-Q., Cai, W., Korre, A., & Ratouis, T. (2022). Induced seismicity associated with geothermal fluids re-injection: Poroelastic stressing, thermoelastic stressing, or transient cooling-induced permeability enhancement? Geothermics, 102, 102404. https://doi.org/10.1016/j.geothermics.2022.102404
- ↑ Mutia, T. M., Fridriksson, T., Magnússon, S. H., & Jónsdóttir, I. S. (2021). Concentrations of sulphur and trace elements in subarctic soils and mosses in relation to geothermal power plants at Hengill, Iceland – ecological implications. Geothermics, 95, 102136. https://doi.org/10.1016/j.geothermics.2021.102136
- ↑ Mott, A., Baba, A., Hadi Mosleh, M., Ökten, H. E., Babaei, M., Gören, A. Y., Feng, C., Recepoğlu, Y. K., Uzelli, T., Uytun, H., Morata, D., Yüksel, A., & Sedighi, M. (2022). Boron in geothermal energy: Sources, environmental impacts, and management in geothermal fluid. Renewable and Sustainable Energy Reviews, 167, 112825. https://doi.org/10.1016/j.rser.2022.112825
- ↑ Bargagli, R., Cateni, D., Nelli, L., Olmastroni, S., & Zagarese, B. (1997). Environmental Impact of Trace Element Emissions from Geothermal Power Plants. Archives of Environmental Contamination and Toxicology, 33(2), 172–181. https://doi.org/10.1007/s002449900239
- ↑ Kimura, H., Shibuya, N., & Kimura, Y. (2012). Hydrogen sulfide is a signaling molecule and a cytoprotectant. Antioxidants & Redox Signaling, 17(1), 45–57. https://doi.org/10.1089/ars.2011.4345
- ↑ Stoppa, G., Nuvolone, D., Petri, D., Centi, L., Nisticò, F., Crocetti, E., Barbone, F., & Voller, F. (2023). Exposure to low levels of hydrogen sulphide and its impact on chronic obstructive pulmonary disease and lung function in the geothermal area of Mt. Amiata in Italy: The cross-sectional InVETTA study. PLOS ONE, 18(11), e0293619. https://doi.org/10.1371/journal.pone.0293619
- ↑ 35.00 35.01 35.02 35.03 35.04 35.05 35.06 35.07 35.08 35.09 35.10 35.11 35.12 35.13 35.14 35.15 35.16 Seyedrahimi-Niaraq, Mirmahdi; Nouri, Tohid (2022). "Investigating the Economic Effects and the Roadmap of Developing Geothermal Systems to Generate Electricity". Journal of Renewable Energy and Environment. Volume 9: pp.52-64.CS1 maint: extra text (link)
- ↑ Cook, David; Davíðsdóttir, Brynhildur; Gunnarsdóttir, Ingunn (2022). "A Conceptual Exploration of How the Pursuit of Sustainable Energy Development Is Implicit in the Genuine Progress Indicator". Energies. 15(6): 2129 – via MDPI.
- ↑ Zhou, Cheng; Doroodchi, Elham; Moghtaderi, Behdad (2013). "An in-depth assessment of hybrid solar-geothermal power generation". Energy Conversion and Management. 74: 88–101 – via Elsevier.
- ↑ Speer, Bethany; Economy, Ryan; Lowder, Travis; Schwabe, Paul; Regenthal, Scott (2014). "Geothermal Exploration Policy Mechanisms: Lessons for the United States from International Applications" (PDF). NREL: pp. 25.CS1 maint: extra text (link)
- ↑ Sahlian, Daniela, Nicoleta; Popa, Adriana, Florina; Cretu, Raluca, Florentina (2021). "Does the Increase in Renewable Energy Influence GDP Growth? An EU-28 Analysis". Energies. 14: 5. line feed character in
|title=
at position 52 (help) - ↑ Xia, Z.H; Jia, G.S; Ma, Z.D; Wang, J.W; Zhang, Y.P; Jin, L.W (December 2011). "Analysis of economy, thermal efficiency and environmental impact of geothermal heating system based on life cycle assessments". Applied Energy. 303 – via Science Direct.
- ↑ Dowling, Alexander; Zheng, Tian; Zavala, Victor (May 2017). "Economic assessment of concentrated solar power technologies: A review". Renewable and Sustainable Energy Reviews. 72: pp.1019-1032 – via Science Direct.CS1 maint: extra text (link)
- ↑ 42.0 42.1 Soltani, M.; Kashkooli, Farshad; Souri, Mohammad; Rafiei, Behnam; Jabarifar, Mohammad; Gharali, Kobra; Nathwani, Jatin (April 2021). "Environmental, economic, and social impacts of geothermal energy systems". Renewable and Sustainable Energy Reviews. 140 – via Science Direct.
- ↑ 43.0 43.1 43.2 Sæþórsdóttir, Anna., Dora; Hall, C., Michael (April 2019). "Contested Development Paths and Rural communities: Sustainable Energy or Sustainable Tourism in Iceland?". Sustainability. 11 (13): 3642 – via MDPI.
- ↑ Óladottir, Oddny (2018). "Tourism in Iceland in Figures" (PDF). ferdamalastofa.
- ↑ 45.0 45.1 45.2 Kamila, Zahratul; Kaya, Eylem; Zarrouk, Sadiq (2021). "Reinjection in geothermal fields: An updated worldwide review 2020". Geothermics. 89 – via Elsevier.
- ↑ Juncu, D.; Árnadóttir, Th.; Geirsson, H.; Guðmundsson, G.B.; Lund, B.; Gunnarsson, G.; Hooper, A.; Hreinsdóttir, S.; Michalczewska, K. (2020). "Injection-induced surface deformation and seismicity at the Hellisheidi geothermal field, Iceland". Journal of Volcanology and Geothermal Research. 391 – via Elsevier.
- ↑ Dhar, Amalesh; Naeth, Anne; Devereaux Jennings; Gamal El-Din, Mohamed (2020). "Geothermal energy resources: potential environmental impact and land reclamation". Environmental Reviews – via Canadian Science Publishing.
|first4=
missing|last4=
(help) - ↑ 48.0 48.1 48.2 48.3 Shortall, Ruth; Davidsdottir, Brynhildur; Axelsson, Guðni (2015). "Geothermal energy for sustainable development: A review of sustainability impacts and assessment frameworks". Renewable and Sustainable Energy Reviews. 44: 391–406 – via Elsevier.
- ↑ Berkes, F. (2007). Community-based conservation in a globalized world. Proceedings of the National Academy of Sciences of the United States of America, 104(39), 15188–15193. https://doi.org/10.1073/pnas.0702098104
- ↑ Finster, Molly; Clark, Corrie; Schroeder, Jenna; Martino, Louis (2015). "Geothermal produced fluids: Characteristics, treatment technologies, and management options". Renewable and Sustainable Energy Reviews. 50: 952–966 – via Elsevier.
- ↑ Harto, C.B; Veil, J. (2011). "Management of water extracted from carbon sequestration projects". Argonne National Lab – via Osti.gov.
This conservation resource was created by Course:CONS200. It is shared under a CC-BY 4.0 International License. |