Course:EOSC270/2023/ Improving Conventional Aquaculture with Geodesic Domes
What is aquaculture?
Aquaculture
Aquaculture is the farming of aquatic organisms, such as fish and algae[1]. The human-controlled process includes breeding, growing and harvesting species. It differs from fishing where seafood is caught, through techniques like trolling and trawling.[2] Aquaculture can be conducted in a diverse array of environments, ranging from ponds to the open ocean, with the majority occurring in South East Asia (>80%).[3]
A range of aquaculture techniques are employed, based on desired species, nutrient input type of water body, price, and productivity. [1]There are four main types as illustrated in Figure 1:
- Floating and Submersible Net Pens and Cages can be installed in any water body and allow for exchange with the natural environment.
- Ponds are artificially enclosed water bodies whose discharge waste can be a serious pollutant if not effectively treated.
- Raceways are flow-through systems where water is diverted to structures stocked with fish and feeding stations.
- Tank cultures are closed systems, allowing for maximum environmental control. [4]
Floating and Submersible Net Pens and Cages | Ponds | Raceways | Tank cultures |
---|---|---|---|
![]() Fish Pens in Chile of Salmon and Mackerel[5] |
![]() Fish Pond Aquaculture Set Up[6] |
![]() Salmon Raceway[7] |
![]() Tank Cultures in Iran[8] |
History and Importance of Aquaculture

Aquaculture’s origins can be traced to ancient civilizations. Modern aquaculture emerged in the 20th century due to technological innovation, population surges, dietary changes and increasing consumer demand for more protein sources, such as seafood. [10]
Aquaculture has several important benefits:
- Increased aquatic organism production allows for more effective resource management as fish stocks can be maintained. Additionally, reliable seafood production helps to alleviate food insecurity as it acts as a nutritious food source.
- Aquaculture provides many economic opportunities, such as through job creation in the farming, seafood processing and equipment production industries as depicted by Figure 2.
- Ocean ranching, a method of restocking the sea with organisms grown in artificially controlled environments, can help replenish and boost the natural fish stock. Ultimately, restoring and supplementing ecosystem health. Moreover, proper farm management and legislation enforcement are essential to prevent overfishing and ecosystem food web destruction.[11]
Coastal vs Offshore Deep Sea Aquaculture
Aquaculture can be performed in many areas, such as along coasts, in the open ocean and in the deep sea.
Conventional coastal aquaculture is a prominent technique in nearshore environments, such as bays and lagoons. The proximity to land allows for a high degree of control regarding breeding conditions. However, the increasing cost and limitation of available coastal locations, public controversy and increasing detrimental environmental effects restrict the development of coastal aquaculture.[12]
Offshore deep sea aquaculture provides new advantages compared to coastal practices. Developments are more resilient and productive as they are no longer limited to coastal space and are no longer subject to harsh surface ocean conditions. Notably, geodesic domes are transportable, submersible open sea cages designed to equally distribute stress leading to increased structural integrity. This technology promotes breeding and protects animals in bad weather leading to higher yields. [13] Open ocean farming can be more environmentally sustainable by preventing the disruption of soil, nutrient levels and benthic communities. Moreover, underwater open ocean aquaculture remains a largely untapped industrial area as seen through the lack of a well established history and government involvement. They are high-risk, but have the potential to be more economically fruitful.[14]
What is the problem with aquaculture?
The design, location and management of the four conventional aquaculture systems has detrimental effects on the surrounding environment. These effects can be categorised by type:
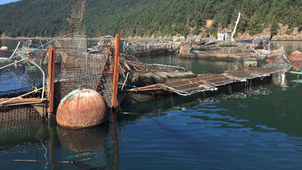
- Design: Weather conditions and poor material quality result in barrier deformations and the breakdown of aquaculture equipment, as seen in Figure 3.[16] The resulting numerically unbalanced interaction between "farm" and "wild" environments has adverse ecological and genetic consequences. Cultivated fish escape into the surrounding natural water bodies and interact with native species.[17] These interactions stimulate the spread of parasites and disease. Lepeophtheirus salmonis and Caligus rogercresseyi are the two main species of sea lice which are transmitted in this manner. Amebic gill disease and the viral pancreas disease are two main players affecting one of the most cultivated fish species, salmon.[18]
- Location: Aquaculture is conventionally located near the coast. Figure 4 shows the distribution of salmon farms along coastlines of the world in 2013. High densities of salmon farms can be seen along the coastlines of Norway, Canada and Chile. These coastlines are home to vulnerable nearshore environments such as mangroves, spawning grounds and seagrass beds. Dissolved organic solids and nutrients from the poorly sited fish farms decrease the water quality of these environments to levels below the threshold. Detailed impacts to the marine environments are described in the section below.[19]
- Management: Fishfeed, without which fish farms would cease to operate, is a leading problem. Roughly 20 million tons of industrial fish, those caught for the sole purpose of creating pellets to feed cultivated fish, are caught each year to sustain aquaculture around the world. Additionally, most aquaculture techniques contribute to environmental pollution by releasing organic waste and plastics into the natural surroundings. Harmful algal blooms, a component of eutrophication, are a resulting consequence. An estimated 35 million tonnes of algae were produced as a result of aquaculture in 2020. The organic matter also covers seagrass beds and blocks sunlight needed by these photosynthesising primary producers. This disturbs the natural ecosystems and has long standing effects on the seafloor and the marine food webs.[20]Figure 4: Distribution of salmon farms along coastlines of the world.[21]
Geodesic domes help resolve a number of these issues through their design and location, detailed in sections below. Management is still a potential area for improvement in conventional and non-conventional aquaculture practices.
Pervasiveness and Prognosis
Approximately half of the world's fish, mollusc and crustacean supply comes from aquaculture. The increase in human seafood requirements drives the increase of aquaculture establishments.[22] China is the leading producer, accounting for 58% of the world's aquaculture produce volume.[23] The regional disparities in production are expected to continue well into the future. It's estimated that marine aquaculture will increase by over 50% by 2050 to meet a global population that is expected to cross the nine billion mark. Molluscs are predicted to remain the most farmed species. Within fish species, finfish is projected to remain the leader thanks to its high protein content and favourable live-to-edible weight ratio. Seaweed production, which requires low manual labour, is a potential area which will develop further in the future. However, if aquaculture is not carried out sustainably, oceans of the world will be impaired beyond a point of no return.[24]
The impacts of conventional aquaculture practices on marine ecosystems?
How does it impact marine ecosystems?
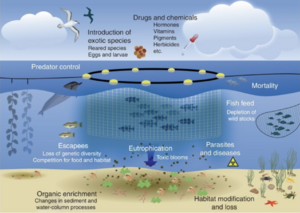
The specific nature of conventional aquaculture practices has resulted in alterations in water quality and destruction of natural habitats due to pollution, the introduction of non-native species into local ecosystems, and an increase in transmission of aquatic diseases among wild and farm marine animals. Figure 6 is a diagram summarizing the interactions and impacts of conventional aquaculture on marine ecosystems.
1. Aquaculture and Virulence amongst marine organisms:
- Stocking densities: Aquaculture stock high densities of farmed organisms in localized areas. Pathogens and parasites thrive best under densely populated conditions such as aqua-farms and therefore, display high infection and mortality rates[26].
- Low genetic diversity: There is limited genetic diversity in aquaculture populations due to selective breeding, the founder effect, and broodstock inbreeding [27]. Broodstock inbreeding particularly refers to the use of a small genetically similar population of fish to establish new populations[28]. Pathogens are highly specialized and tend to be unique to specific marine species. An absence of genetic variability therefore, increases virulence and mortality rates [26].
- Translocation: Fish pens and cages are susceptible to damage due to unpredictable storms, currents and predators [26]. As a result, large numbers of marine organisms have escaped their fish pens, increasing the risk of parasite exchange among wild marine ecosystems. The spread of disease places enormous risk on marine specie survival and population densities.
2. Introduction of Invasive Species:
High probability of escape associated with open-fish pens and cages indicates greater risk for the introduction of invasive species into marine ecosystems. These invasive species swim into the open ocean and compete for shelter and food with other wild marine populations altering marine food webs and increasing mortality rates for many marine organisms [29]. A disastrous flooding event released non-native asian carp imported from South America into the Mississippi river where these invasive species started to outcompete native fish for food and space, shifting marine ecosystems and decreasing native fish populations. Such fish populations included yellow perch, blue gill and white crappie fish[30].
3. High fish feed demand:
Fin-fish such as salmon, trout, cod, and tuna are carnivorous and require a large supply of wild fish stock to meet growth demands (anchovies, herring, sardines). These foraged fish are prey to many other wild marine organisms such as whales, larger fish, sea birds and other marine mammals [31]. The process of extracting and foraging wild fish stock for aquaculture depletes wild marine predators of their resources, deteriorating many wildlife populations [31].
4. Destruction of natural habitats:
Ecosystems dense with primary producers important for supplying energy to marine food chains such as mangrove forests, coastal reefs, and estuaries are destroyed to create ponds for fish farms. High demand for shrimp aquaculture in Asia and South America, is the leading cause of mangrove forest deforestation in Asia and South America[32]. As autotrophic organisms, many primary and secondary consumers rely on the availability of mangroves for energy and shelter. Mangrove deforestation therefore, increases marine life mortality and alters the food web[32].
5. Water Quality
Open-net fish pens and cages release untreated compounds (farm feces, uneaten nutrients, pesticides, and antibiotics) directly into the surrounding ecosystem, impacting sediment metabolism (increase release of sulfide and methane bi-products)[33], enhancing toxic algal bloom, depleting oxygen levels and decreasing redox potential[31]. These alterations are not suitable for the survival of highly sensitive sea-bed and benthic communities[31]. In coastal regions, the prevalence of toxic sedimentation for longer durations can lead to the mortality of sedentary fauna populations, especially suspension feeders [34]
Incorporating geodesic domes as an alternative aquaculture model can improve the aforementioned negative impacts conventional aquaculture has on the environment.
Given the impact, what are the solutions?
What is the alternative?
Addressing the ecological impacts due to conventional aquaculture have proven to pose a significant challenge; however, changes that can have the most impact on the quality and quantity in the production of aquaculture are location and pen designs. When looking at alternatives to conventional methods, one of the most promising developments is moving to the open ocean. Although three of the four types of aquaculture (inland, freshwater, and coastal) have limited viable space and possess extensive environmental and ecological footprints,[35] deep sea aquaculture has the potential to solve or at the very least mitigate the majority of ecological and environmental concerns that are brought up with the industry.[36]
Viability of Deep Sea Aquaculture

In its simplest terms, the ocean contains two areas, places where biological productivity and net primary production thrive, and places where they do not. As indicated in Figure 7, concentrations of net primary production predominantly occur near the continental shelves, meaning that the larger part of the ocean is relatively uninhabited by net primary production and benthic plants, which are at the base of every ecosystem impacted. These areas in which concentrations of primary production begin to fall off is where aquaculture gains a high ceiling of development.[36]
The open ocean holds a relatively untapped and extensive surface area where farming operations can be held with minimal disturbances to species and damage to the surrounding environment. The sheer size and depth of the open ocean offers a more stable and less variable environment than nearshore waters, which are often subject to storms, pollution, and other environmental factors that can complicate operations and fish welfare.[36] Alongside this, it leaves little room for habitat degradation and water quality damage caused by farming practices since there is no habitat other than the open water tens of kilometers offshore with depths in the hundreds of meters. Its abiotic features contribute to controlling issues such as filtration and waste management since there is high circulation due to the ocean currents and time for waste to be naturally absorbed and distributed in the surrounding environment.[37]
Development of Geodesic Dome Designs
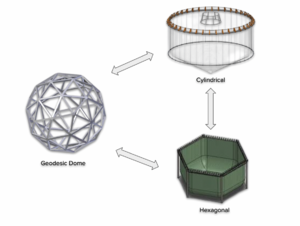
Aquaculture farms have predominantly used cylindrical or hexagonal pen designs; however, from an economic and ecological perspective, both designs are relatively inefficient. Structurally, they both can not distribute stress as effectively throughout the structure, making it more susceptible to harsh conditions without proper reinforcement. They also possess a limiting factor in terms of the surface area to volume ratio, meaning that it is not the most efficient use of space, hindering production and increasing the footprint of the farm. Ecologically, these designs cause many issues, as their implementation often comes with being managed overcapacity and improper filtration in an effort to keep up with demand.[39]
This is where the development of a geodesic dome design comes in, as the benefits include the structural integrity and efficiency of the dome when used in tandem with deep sea aquaculture. The interconnected triangles distribute stress throughout the structure, making it resistant to harsh conditions such as the strong currents, high winds, and extreme pressure. This feature makes it an ideal choice for operations, as it can withstand the unpredictable ocean environment. In terms of maximizing space, the dome’s shape provides the highest surface area to volume ratio, making it an efficient use of space which maximizes production while minimizing the overall footprint of the farm.[40]
How do geodesic domes improve on current aquaculture designs?
Geodesic domes as an alternative practice
Geodesic domes are viewed as alternative to these methods, and as a solution to some of the problems they create. Its main premise is growing and housing fish in the open ocean, to leave a very minimal environmental impact, in stark contrast to some of the current aquaculture designs[40]. These domes are huge, semi-submersible, and modular structures, typically made up of triangles and put together to form a spherical-type shape. As a result, they are very strong systems, with the ability to withstand the force of ocean currents/the tide[39].
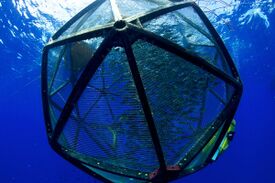
The advantages of these geodesic domes stems mostly from their shape, and the location in which they are placed (the open ocean). The geodesic shape results in a high surface area to volume ratio, the ability to flow with ocean currents, thus causing less disturbance on the cage and its fish, and also the ability to transport the domes with relative ease between different marine locations. [42]These benefits aid in reducing impacts on coastal environments, and crucially reducing fish mortality[43]. Furthermore, these geodesic domes can be internally controlled quite well, with the potential to change water temperature through solar heating systems, which is seen in some of the most modern designs[44]. This idea of control over the interior of the dome is one that is critical in not only managing the output of fish stock, but also ensuring the fish are kept in much better condition.
La Paz, Mexico
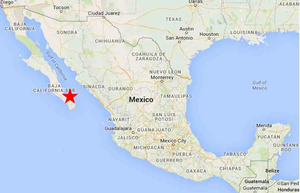
La Paz is a coastal city in the Mexican state of Baja California Sur, West Mexico. 2 miles off the coast, an aquaculture system, consisting of open-ocean geodesic domes has been created. This system has an area of around 3.5 km2, and goes to depths of 30-45 metres below the ocean surface. Sustainability is at the heart of this project, with the Totoaba species, one that is endemic to the Gulf of California and has been deemed endangered, needing protection by the team which started this operation[46]. Since the implementation of this more sustainable approach to aquaculture, Totoaba populations have increased, and a more sustainable practice of using increased soya protein as their protein source, instead of fishmeal, has been enacted. In turn, this not only has beneficial environmental impacts, it also reduces production costs and thus consumer price[47], which is extremely pivotal given the challenging economic times the entire world is living through right now.
References
- ↑ 1.0 1.1 Pullin, Roger; Sumaila, Rashid (2005). "Aquaculture". Fish for Life: Interactive Governance for Fisheries: 93–108 – via JSTOR.
- ↑ Bardach, John E. (1968). "Aquaculture". Science. 161: 1098–1106 – via JSTOR.
- ↑ "Aquaculture". The Canadian Encyclopedia. April 2013.
- ↑ "Aquaculture methods". SeaChoice. May 2021.
- ↑ Leggett, Gordon (16 March 2019). "Aquaculture in Chile".
- ↑ Korrigan, Paolo (29 September 2017). "Fish Pond (Aquaculture)".
- ↑ Cheri, Anderson (27 February 2013). "Service hatchery staff crowd juvenile salmon out of a raceway".
- ↑ Movahedinejad, M.Hossein (8 May 2019). "Fish farming in Iran".
- ↑ @JopkeB (22 May 1991). "Seafood stall Barcelona, near the Rambla".
- ↑ Amundson, Clyde; von Brandt, Andres (December 2022). "Aquaculture". Encyclopedia Britannica.
- ↑ "Aquaculture supports a sustainable earth". NOAA. September 2022.
- ↑ Sanchez-Jerez, P., Karakassis, I., Massa, F., Fezzardi, D., Aguilar-Manjarrez, J., Soto, D., Chapela, R., Avila, P., Macias, J. C., Tomassetti, P., Marino, G., Borg, J. A., Franičević, V., Yucel-Gier, G., Fleming, I. A., Biao, X., Nhhala, H., Hamza, H., Forcada, A., & Dempster, T. (2015). Aquaculture’s struggle for space: the need for coastal spatial planning and the potential benefits of Allocated Zones for Aquaculture (AZAs) to avoid conflict and promote sustainability. Aquaculture Environment Interactions, 8, 41–54. https://www.jstor.org/stable/24864967
- ↑ S. Muthukumarvel, A. Kishor, V. Gowthaman, G. Dinesh and T. Sudhakar, (2022) "An Assessment of technologies requirements in Open sea cage farming for Indian sustainable Aquaculture," OCEANS 2022 - Chennai, Chennai, India, pp. 1-7, doi: 10.1109/OCEANSChennai45887.2022.9775309.
- ↑ Soto, Doris; Wurmann, Curtis (2019). "Offshore aquaculture: A needed new frontier for farmed fish at sea". The Future of Ocean Governance and Capacity Development: 379–384.
- ↑ King 5 News. (2022). Thousands of fish escape from a Cooke Aquaculture fish farm off the West Coast near Victoria, B.C. Global News. Retrieved March 8, 2023, from [1]
- ↑ Sievers; et al. (20 September 2021). "Assessment of challenges and opportunities for this technology offers opportunities to improve, expand commercial fish production". Retrieved 8 February 2023. Explicit use of et al. in:
|last=
(help) - ↑ Jensen; et al. (12 August 2010). "Escapes of fishes from Norwegian sea-cage aquaculture: causes, consequences and prevention" (PDF). AQUACULTURE ENVIRONMENT INTERACTIONS. 1: 71–83. line feed character in
|title=
at position 42 (help); Explicit use of et al. in:|last=
(help) - ↑ Lekang; et al. (23 March 2016). "Challenges and emerging technical solutions in on-growing salmon farming". Aquaculture International. 24: 755–766. Explicit use of et al. in:
|last=
(help) - ↑ "Habitat". Retrieved 8 February 2023.
- ↑ Cole; et al. (July 2009). "Aquaculture: Environmental, toxicological, and health issues". International Journal of Hygiene and Environmental Health. 212-4: 369–377. Explicit use of et al. in:
|last=
(help) - ↑ GIS Team. (2013). Coastlines of the World Affected by Salmon Farms. Living Oceans Society. Retrieved February 8, 2023, from [2]
- ↑ "Aquaculture accounts for half of the world's fish supply". Retrieved 8 February 2023.
- ↑ Naylor; et al. (24 March 2021). "A 20-year retrospective review of global aquaculture". Nature. 591: 551–563. Explicit use of et al. in:
|last=
(help) - ↑ "Marine Aquaculture Forecast". Retrieved 8 February 2023.
- ↑ Beddows, Caitlin (16th February 2016). "Restoring Coastal Ecosystems from Fisheries and Aquaculture Impacts". Retrieved 7th March 2023. Check date values in:
|access-date=, |date=
(help) - ↑ 26.0 26.1 26.2 Bouwmeester, Mark.M (11 October 2020). "Collateral diseases: Aquaculture impacts on wildlife infections". Journal of Applied Ecology.
- ↑ Kennedy, David (27 October 2015). "Potential drivers of virulence evolution in aquaculture". Evolutionary Applications.
- ↑ https://www.merriam-webster.com/dictionary/brood%20stock#:~:text=noun,game%20birds)%20in%20suitable%20habitats. Missing or empty
|title=
(help) - ↑ Ju, Rui-Ting (9 October 2019). "Emerging risk of non-invasive species escapes from aquaculture". Applied Ecology.
- ↑ Flesher, John (20 November 2019). "Study links Asian carp with Mississippi River fish drop".
- ↑ 31.0 31.1 31.2 31.3 Okumus, Ibrahim. "Coastal Aquaculture: Sustainable development, resource use and integrated environmental management". Turkish J Marine Sciences.
- ↑ 32.0 32.1 Ahmed, Nesar (21 January 2016). "Coastal aquaculture, mangrove deforestation and blue carbon emissions: Is REDD+ a solution?". Science Direct.
- ↑ Sanz-Lazaro, Carols (15 December 2021). "The environmental effect on the seabed of an offshore marine fish farm in the tropical Pacific". Journal of Environmental Management. 300.
- ↑ Gaspar, M.B (2011). Treatise on Estuaries and Coastal Science: Restoring Coastal Ecosystems from Fisheries and Aquaculture Impacts. ISBN 978-0-08-087885-0.
- ↑ Sanchez-Jerez, P. (January 6 2016). "Aquaculture's struggle for space: the need for coastal spatial planning and the potential benefits of Allocated Zones for Aquaculture (AZAs) to avoid conflict and promote sustainability" (PDF). Aquaculture Environment Interactions. Retrieved 8 March 2023. Check date values in:
|date=
(help) - ↑ 36.0 36.1 36.2 36.3 Boyd, Philip (January 2014). "Net Primary Production in the Ocean" (PDF). Cross-Chapter Box. Retrieved February 8 2023. Check date values in:
|access-date=
(help) - ↑ Holmer, Marianne (25 February 2010). [www.int-res.com/articles/aei2010/1/q001p057.pdf "Environmental Issues of Fish Farming in Offshore Waters: Perspectives, Concerns, and Research Needs"] Check
|url=
value (help) (PDF). Aquaculture Environment Interactions. - ↑ Ad.Aq (March 2023). "Cages". Advanced Aquaculture. Retrieved 8 March 2023.
- ↑ 39.0 39.1 Smith, Peter (08/09/2012). "A Gentler Fish Farm, Powered By A Geodesic Dome". Fast Company. Retrieved 01/02/2023. Check date values in:
|access-date=, |date=
(help) - ↑ 40.0 40.1 "Geodesic Domes For Deep Sea Fish Farming". Geodomas. Retrieved 01/02/2023. Check date values in:
|access-date=
(help) - ↑ Richard, Hires. "Hydroponic Farming".
- ↑ McCormack, Craig William; Phillips-Hungerford, John Taylor (2019). "A dangerous precedent; the geodesic dome as a credible space architecture typology" (PDF). 70th International Astronautical Congress, IAC – via International Astronautical Federation.
- ↑ Muthukumarvel, S.; Kishor, Anand; Gowthaman, V.; Dinesh, G.; Sudhakar, Tata (2022). "An Assessment of technologies requirements in Open sea cage farming for Indian sustainable Aquaculture". OCEANS 2022: 1–7.
- ↑ Fuller, R.J. (2007). "Solar heating systems for recirculation aquaculture". Aquacultural Engineering. 36: 250–260.
- ↑ On The Road in Mexico (2019). "La Paz, Baja California Sur". On The Road In Mexico. Retrieved 02/03/2023. Check date values in:
|access-date=
(help) - ↑ "EOF TEAM". Earth Ocean Farms.
- ↑ Davila-Camacho, Claudia; Galaviz-Villa, Itzel; Lango-Reynoso, Fabiola; Castaneda-Chavez, Maria del Refugio; Quiroga-Brahms, Cecilia; Montoya-Mendoza, Jesus (2018). "Cultivation of native fish in Mexico: cases of success". Reviews in Aquaculture. 11: 816–829.