Genetic Assimilation
Genetic assimilation is when a character, originally called an acquired character due to the fact they were produced because of the environment, has now produced a phenotype. Natural selection is the mechanism used to produce this new phenotype. The new phenotype no longer requires the original stimulus to be observed. It was once believed that acquired characters follow Mendelian inheritance, however further studies have proven that they are not inherited but rather developed as the result of environmental adaptation[1].
Genetic assimilation is also known as “phenotype precedes genotype” evolution. This means that a new morphology may arise because of a consistent response to an environmental stimulus. The morphology is reinforced later on to be present in the absence of the environmental stimulus due to the evolution of its genetic causes. The environmentally induced conditions do not result in the formation of genetic causes, but rather forces mutations favored by natural selection. These mutations support the phenotype to adapt to the environmental stimulus, and is not Lamarckism[2].
A developmental pathway of a trait occurring without an environmental stimulus results in a graph depicting phenotypes in a bell shaped curve. This can be understood as traits being dependent on their genotype. However, when a pathway has a strong influence from canalization, the appearance of a trait is not dependent on the genotype. All but the extremes will look the same. Despite all the genotypes looking the same the organisms will not react the same under certain conditions. Genetic assimilation determines the normal outcome under certain environmental stimuli, also known as phenotypic plasticity.
Phenotypic plasticity is not an evolutionary stage,rather it is a developmental process. Although it is not an evolutionary stage, it does lead to an evolutionary process. Under the right circumstances phenotypic plasticity is the target of natural selection , which is an evolutionary process. As a result of the selection, genetic assimilation is observed and an evolutionary outcome is produced.
Conrad Hal Waddington produced one of the most well known experiments providing evidence for genetic assimilation. In his experiment he used Drosophila melanogaster and focused on their wings, specifically the organisms that were crossveinless. He found that when the larvae Drosophila were exposed to a heat shock it was born crossveinless[3] . He conducted another experiment where he studied larvae Drosophila that had been exposed to ether vapor. This exposure caused them to produce another thorax, also known as bithorax organisms[3]. In the end it was noted that after many generations the artificially selected trait was present without the stimulus, whether it was a temperature shock or ether vapour.[4] A more recent study done by Dr. Richard Palmer also shows strong evidence of genetic assimilation. His research was based on bilateral asymmetry in a variety of taxa as well as studying the canalization of the vertebrate heart[2].
Key Concepts
Phenotypic accommodation is adaptive adjustment of variable aspects of an organism’s phenotype during development without genetic change[3]. It is the result of adaptive developmental responses, so the resulting morphologies are not random; instead they represent past functionality. Phenotypic accommodation can facilitate the evolution of morphology by removing the negative effects of change. It can be induced by a mutation or environmental factor, both of which come from ancestral developmental responses rather than from the inducing factor itself.
The Baldwin effect, also known as ontogenic evolution, and is a theory about a mechanism for specific selection for learning[3]. The theory states that certain offspring have a better capacity for learning, and emphasizes that these members of a species can shape the evolution of that species.
Canalization is a way of measuring the ability of a population to produce the same phenotype regardless of variation in its genotype or environment[3]. It is brought up when developmental pathways are shaped by evolution. This idea was brought about by C. H. Waddington, who suggested that developmental reactions are adjusted to create a definite end result without regard to variable conditions.
Novel phenotypes may be expressed under environmental stimulus due to cryptic genetic variation. These are traits that are not usually expressed, but may be a source for new phenotypes under certain environmental conditions [5].
Stabilizing selection is a form of natural selection where the amount of genetic diversity in a population decrease as the population stabilizes on a particular trait. It is thought of as the most common mechanism for natural selection, because it regularly uses negative selection, which selects against uncommon traits, therefore reducing phenotypic variation.
Mechanisms
Mutations are thought to be the main source of variations in organisms. Gene assimilation is a not a form of mutation but rather a change in phenotype. In order for gene assimilation to take place there must be some mechanisms to cause the this change. The first mechanism for gene assimilation is in the absence of an environmental change where a cryptic trait is not expressed since an environmental threshold has not been reached. When an environmental change occurs and the threshold is reached this cryptic trait is expressed. Due to phenotypic plasticity and phenotypic accommodation, the ability to adjust phenotypic traits very quickly, theses phenotypic changes can be happen very quickly[1]. With this different environment the new phenotype is selected for by natural selection and the old trait is selected against and will eventually disappear. Once this trait reaches an even higher threshold the trait will become permanent, even though the environmental stimulus is gone. This directional selection to the new trait is called canalization.
Canalization is where a phenotype is no longer plastic and it moves in directional selection towards the new phenotype independently of environmental conditions where it will eventually stabilize,Stabilizing selection[6][7]. Canalized traits are difficult to alter under environmental conditions since they are considered to be optimal traits. These traits are also thought to be the most crucial to fitness. It is thought to be a buffer against mutations. Genetic assimilation is also responsible for genetic fixation.
Genetic Accommodation
Genetic assimilation is a type of genetic accommodation. In 2003 Mary Jane West-Eberhard proposed that “Genetic assimilation is a special case of a more general phenomenon, called genetic accommodation”[8]. West-Eberhard goes on to describe genetic accommodation as a three step process similar to genetic assimilation but has a few key differences. In genetic assimilation the trait becomes fixed and is no longer plastic. This means that the trait is no longer sensitive to the environment, environmental canalization, and will undergo natural selection. While in genetic accommodation the trait can become fixed, like in genetic assimilation, or it can remain plastic therefore it is able to revert to the old phenotype. Also they differ by what causes the change in phenotype. Genetic assimilation states that an environmental trigger causes a change in phenotype. In genetic accommodation an environment trigger can cause a change in phenotype also a genetic trigger can cause a phenotypic change. Not only can a change be phenotypic it can also be behavioural.
Behavioural Asymmetry
It is sometimes difficult to distinguish what is behaviour and what is morphology. Many behavioural traits are due to morphology, for instance sneaker male cuttlefish. They sneak because of a morphological trait even though it appears to be behavioural. [9] . In 2010 Hinchliffe and Palmer did a study on caddisfly ,Trichoptera, larvae and the difference in cases among the species. They found that species that formed helicospiral cases, which mimic snail shells, were less likely to be eaten by fish as opposed to species that formed spiral-walled elongated tubes. This type of behavioral mimicry is seen in a few species in the Trichoptera order. It is thought that some species use helicospiral cases to make them resemble small snail to confuse fish since fish would rather eat a small arthropod as opposed to a small snail.
Waddington's Experiments
Conrad Hal Waddington was an English biologist who carried out multiple experiments in the interest of multicellular development in embryos from an evolutionary standpoint. His experiments demonstrated that evolutionary change was able to take place without the action of selection on spontaneous mutations, suggesting that Lamarckian mechanisms were coming into play. The basis for Waddington’s experiments was a select strain of Drosophila melanogaster with a select character. There was no apparent adaptive function for this select character, as it did not appear under normal circumstances, and therefore was chosen to be observed to see if the trait could become genetically assimilated if favored by selection.
When a select strain of Drosophila melanogaster was given a temperature shock of 40⁰C for four hours, at 17-23 hours after puparium formation, a fair number of crossveinless wings appeared. [3].After choosing to concentrate on this cross-veinless wings effect, two separate selection lines were set up. In one, only those flies which showed the crossveinless effect were bred from, which created “upward selection”. In the other, the crossveinless flies were rejected, and those with normal wings were bred, creating “downward selection.” Flies were classified as “crossveinless” when they showed disruption in the posterior crossvein.
In the first few generations, the response to selection was somewhat irregular, although downward selection showed some signs of being effective. It was then discovered that the most effective time frame for temperature shock was between 21 and 23 hours, and after this discovery selection proceeded rapidly in both directions. No crossveinless individuals were found until generation 14, and in generation 16 the population was about 1-2% crossveinless.[10] It was then noted that the intensity of the temperature shock required to cause the mutation to appear began falling from one generation to the next. This was an extremely interesting discovery, as it showed the beginning of the genetic assimilation of crossveinless wings in the flies.
In a second experiment[11], using the same select Drosophila melanogaster, Waddington exposed the larvae to ether vapour, which resulted in a bithorax mutation. In this mutation, the meta-thoracic disk gives rise to structures which normally define the mesothorax. Once again, Waddington created two different selection lines; the first was flies which showed the bithorax phenotype, and the other was normal wild-type flies. The methods used in the second experiment generally followed the same procedures as the first crossveinless experiment – using an environmental shock to induce a selected phenotype, and then gradually decreasing the intensity of the shock in order to try and cause the selected phenotype to breed true within a select lineage.
There were three major outcomes from these experiments:[12]
1) The proportion of adults which displayed the desired phenotype continued to rise from one generation to the next
2) The flies began to increasingly resemble the mutant type
3) The environmental shock required to induce a phenotypic change decreased from generation to generation.
From the evidence produced by these experiments, Waddington proposed a very simple explanation.[13] He stated that if an individual possesses a heritable trait, it is likely that close relatives of the individual will carry the gene, regardless of whether it is expressed or not. This principle is known as sib-selection. Sib-selection explained why even though many of the later generations were not even exposed to the environmental shock, they still began to express the selected character. In the natural world, the selected character, whether it is crossveinless wings or a bithorax, is not beneficial to the individual, and therefore is not often expressed, despite the fact that the individual still carries the gene and has the ability to express it. This buffering action is referred to as canalization. In a laboratory setting, this buffer effect does not come into play, as the non-adaptive traits are artificially selected for. This results in the expression of genes which would not normally be expressed, which is the basis for genetic assimilation.
Symmetry Breaking
Research on bilateral asymmetry by Dr. Richard Palmer is some of the strongest evidence to date supporting genetic assimilation. Bilateral asymmetry is an ideal trait to study because it has evolved multiple times independently in a multitude of different taxa and has a simple binary-switch nature [2]. This makes a variety of different organisms easy to compare. To understand the processes of genetic assimilation in symmetry breaking, one must first understand axes development in bilateral organisms. They are composed of four, rather than three body planes: anteroposterior, dorsoventral, and separate left and right mediolateral axes. There is not a single left-right axis, but rather two mirror-image mediolateral ones. Following the development of the anteroposterior and dorsoventral axes, the default state of the organism is bilateral symmetry, as the left and right axes are mirror images. This implies that a symmetry-breaking step (mutation or developmental factor) must be involved for bilateral asymmetry.
There are two types of bilateral asymmetry: antisymmetry and directional asymmetry [2]. In antisymmetric organisms the dextral and sinistral forms are equally frequent, and the direction of asymmetry is almost never inherited . In directionally symmetric organisms, most organisms are asymmetric in the same direction, and the direction of asymmetry is heritable. Novel phenotypes can arise through both mutations and environmental factors during development (developmental plasticity). These two sources of novel traits correspond to two evolutionary mechanisms.
In the conventional mode of evolution, the organism is originally symmetric and mutation and selection cause directional asymmetry (a). In the alternate mechanism, a mutation causes asymmetry without directional bias. The organism now has three phenotypes: symmetric (wild-type), and sinistral/dextral (mutant). Upon fixation, the antisymmetric individuals (mutants) persist (first leg of b). The second stage corresponds to genetic assimilation as a non-heritable antisymmetric phenotype becomes genetically heritable through random mutations. The phenotype becomes genetically canalized (second leg of b)[2].
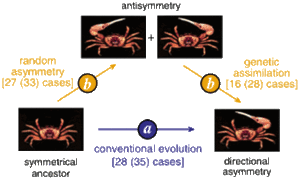
Roughly half of the cases followed the genetic assimilation mechanism rather than conventional evolution. However, genetic assimilation may be even more prevalent. Once a trait has become hereditary, it is difficult to prove that it was once due to developmental plasticity. If there are no known antisymmetric taxa, then genetic assimilation cannot be inferred. This is known as ‘ghosts of genetic assimilation past’ and may be more prevalent than formerly believed.[2]
Heteranthera
In one compelling case, direction of asymmetry is heritable in antisymmetric heteranthera plant species. Heteranthera produces enantiostylic flowers, that is, the style is deflected to either the right or left side. Flower types are either monomorphic, where either style type are found on the same individuals, or dimorphic, where the style direction is genetically fixed.[14] In monomorphic plants, direction of the style is thought to be controlled by environmental factors. For dimorphic individuals, dextral or sinistral form is controlled by a single biallelic locus, with right being dominant to left [14]. Dimorphic types are less common and more derived than monomorphic types. This implies that asymmetric phenotypes arose before the biallelic gene. How this gene arose is unknown.
Canalization of the Vertebrate Heart
All living vertebrates have a heart that is conspicuously displaced towards the left. Despite the conserved end result, the developmental mechanisms differ substantially between different orders. Spontaneous directional change of the heart is possible in individuals throughout all vertebrates. More primitive organisms have a higher rate of spontaneous reversal: 5% in fish and 2% in amphibians, declining to about 0.1% in mammals and 0.01% in humans.[2] This implies that through evolution, the direction of the vertebrate heart has become more canalized. This increase in canalization may be due to different developmental mechanisms or the environmental conditions during development. In mammals, symmetry breaking is determined by cilia directing the Nodal signaling protein leftward in the embryo. Mammals are also live-bearing organisms; early development occurs within a predictable environment. Changes in the external environment are less likely to influence the rate of spontaneous reversals in mammalian species.[2] Whether canalization is due to differences in developmental mechanisms or the developmental environment is unclear.
Controversies
Many scientists have not yet accepted genetic assimilation to be a crucial part of evolution. There are many different reasons about different aspects of genetic assimilation that cause doubt. One of the arguments against the importance of genetic assimilation is related to phenotypic plasticity. Some scientists think that phenotypic plasticity is not important primarily because they believe it to only be an intermediate stage of genetic assimilation. This thought is falsified because phenotypic plasticity is essential for survival of the organism under the new environmental stimulus. If this assumption were to be believed the result would be a loss of phenotypic plasticity as well as genetic assimilation occurring without natural selection[1].
Phenotypic plasticity is also involved in the second argument. This one is about how adaptation aspect of phenotypic plasticity cannot be predicted from quantitative genetic models. Some think that phenotypic plasticity can be predicted, however, this is not true. Genetic models cannot predict phenotypic plasticity because there are several common factors between multiple different models. These similarities show that outcomes of different pathways of evolution cannot be distinguished from each other[1]. Phenotypic plasticity is not an alternative from of natural selection. Natural selection simply acts on developmental plasticity.
The final and most prevalent argument against genetic assimilation is that there is a lack of evidence and convincing examples of when and how it occurs. This is because genetic assimilation can occur very quickly as it only needs a few generations to occur. Genetic assimilation could thereby go by unnoticed, especially if you are not looking for it. Another reason is that evolutionary biology is a historical science, meaning it requires a hypothesis in order to be tested. Although there are no hypotheses testing for or against genetic assimilation, there are many studies that show that genetic assimilation is an active area of theoretical inquiry. Currently there have been a few experimental studies, with the possibility of many more being conducted in the future[2].
References
- ↑ 1.0 1.1 1.2 1.3 Pigliucci, Massimo, Courtney J. Murren, and Carl D. Schlichting (2006). "Phenotypic plasticity and evolution by genetic assimilation". The Journal of Experimental Biology. Cite journal requires
|journal=
(help)CS1 maint: multiple names: authors list (link) - ↑ 2.0 2.1 2.2 2.3 2.4 2.5 2.6 2.7 2.8 Palmer CH (2004). "Symmetry Breaking and the Evolution of Development". Science. 306 (5697): 828–833.
- ↑ 3.0 3.1 3.2 3.3 3.4 3.5 Waddington C.H. (June 1953). > "Genetic Assimilation of an Acquired Character". Evolution. Cite error: Invalid
<ref>
tag; name "Waddington" defined multiple times with different content - ↑ Waddington C.H. (October 1952). "Genetic Assimilation of an Acquired Character". Society for the Study of Evolution.
- ↑ Dworkin, Gibson CH (2004). "Uncovering cryptic genetic variation=Nature". 5: 681–690. Cite journal requires
|journal=
(help) - ↑ "Symmetry breaking and genetic assimilation – Pharyngula." ScienceBlogs - Where the world turns to talk about scienc. 2006
- ↑ Meiklejohn, Colin D. , and Daniel L. Hartl. "A single mode of canalization." Trends in Ecology and Evolution 2002
- ↑ Braendle, Christian, and Thomas Flatt. "A role for genetic accommodation in evolution?." Bioessays 2006): 268-273. what the paper says. Web. 24 Nov. 2012
- ↑ Hinchliffe, Robert , and A.R. Palmer. "Curious Chiral Cases of Caddisfly Larvae: Handed Behavior, Asymmetric Forms, Evolutionary History." Integrative and Comparative Biology 2010.
- ↑ Waddington C.H. (June 1953). > "Genetic Assimilation of an Acquired Character". Evolution.
- ↑ Vidyanand Nanjundiah (June 2010). > "C H Waddington, Canalisation and Genetic Assimilation" Check
|url=
value (help). Resonance. - ↑ Vidyanand Nanjundiah (June 2010). > "C H Waddington, Canalisation and Genetic Assimilation" Check
|url=
value (help). Resonance. - ↑ Erika Crispo (June 2007). > "THE BALDWIN EFFECT AND GENETIC ASSIMILATION: REVISITING TWO MECHANISMS OF EVOLUTIONARY CHANGE MEDIATED BY PHENOTYPIC PLASTICITY" Check
|url=
value (help). Evolution. - ↑ 14.0 14.1 Jesson CH (2002). "The genetics of mirror-image flowers". Biological Science. 269 (1502): 1835–1839.