Course:PATH417:2021W2/Case2
Path 417 Cases |
---|
Instructor |
David Harris
Agatha Jassem Ramon KleinGeltink Inna Sekirov |
Case Projects |
Case 1 |
Case 2 |
Case 3 |
Case 4 |
Case 2
Suzanne is a 24-year-old avid hiker and excited to visit her friends in Halifax for some hiking in some new scenery. When she arrived on the plane from Vancouver, it was a sunny spring day in Halifax and her friends wanted to show her a great view of the city. Her friends picked her up at the airport and they headed to the nearby Admiral’s Cove for a scenic hike to the top of a ridge overlooking the Halifax Harbour. That evening they had a wonderful night out visiting multiple local craft breweries and tasting east coast lobster. The next morning Suzanne noticed a non-painful bump on the back of her calf, but she was on the way out with friends for another hike, so she did not pay much attention to it. On the hike, as Suzanne was climbing up a steep area, one of Suzanne’s friends behind her noticed the back of Suzanne’s leg and asked to look at it. It was a tick, and it had embedded its head into the back of Suzanne’s leg. Her friend removed the tick and they carried on. Over the next few days, Suzanne developed a ring-like red rash at the site of the tick bite. The rash was hot to the touch and felt like it was burning. Suzanne felt chills and fatigued. Knowing that Lyme disease was in the area, her friend took Suzanne to an Urgent Care Centre (UCC) for assessment. At the UCC, Suzanne head a temperature of 38.0 degrees Celsius, a white blood cell count of 14, and the physician examining her said her rash was a classic “bull’s eye” rash caused by Borrelia burgdorferi and prescribed her antibiotics for erythema migrans.
1. The Body System
Question (i): Describe the signs and symptoms presented in the case. Are there any other signs or symptoms that could have been commented on but are not presented in the case? What are the key History of Presenting Illness elements presented? What laboratory samples are taken and why? What are the meanings of the laboratory results reported? (No need to describe physiology of the signs and symptoms and no need to describe the laboratory testing itself as these are the basis of other questions).

Lyme disease is caused by the bacterial spirochete pathogen Borrelia burgdorferi (B. burgdorferi) (1). The vector for Lyme disease are various types of hard-bodied ticks, such as the western black-legged tick (Ixodespacificus) found on the pacific coast and the black-legged tick (Ixodesscapularis) found on the eastern coast of Canada and the United States. The ticks are responsible for the transmission of B. unitedurgdorferi to the host upon biting and attachment to the skin(1). These black-legged ticks are also found in Nova Scotia, where Suzanna was going on her hike.
In a medical context, the word "sign", as derived from the Latin word "signum", refers to a manifestation of the disease that can be objectively identified by another individual, usually by a physician in the clinic or at the bedside (2). The first sign noticed in the case was the non-painful bump noticed on her leg. The signs described in this case include the non-painful bump on the back of Suzanne's calf, the "bull's eye" rash that developed over a few days, as well as the heat felt upon touching the rash. All of these indicators can be objectively identified by another individual (i.e., Suzanne's friend), and, therefore, are considered signs. The "bull's eye" rash, in particular, is a skin lesion known as erythema migrans, and it is the most common sign associated with Lyme disease (2). It usually develops at the site of the tick bite within 1-2 weeks (3). The patient also has a fever with a head temperature of 38.0 ℃; most commonly, a head temperature of 38.0 ℃ and higher is considered a fever (1,4). Because the temperature was measured objectively by a healthcare professional, Suzanne's fever is considered a sign.
The word "symptom", on the other hand, is derived from the Greek word "symptoma", and it refers to a subjective experience that can only be identified by the patient themselves (2). Suzanne’s symptoms (experienced characteristics) include chills, fatigue, and a burning sensation at the site of her rash. However, a few symptoms were not referenced that could be present early in disease manifestation, Influenza-like symptoms such as headache, body aches, neck stiffness, sore throat, swollen lymph nodes as well as other symptoms within one week could be malaise and regional lymphadenopathy; all which depict early disease manifestation of Lyme disease (5,6).
The history of present illness (HPI) provides the information necessary to generate a differential diagnosis (7). Suzanne’s key history finding is that she was bitten by a tick recently, prior to feeling these signs and symptoms. Ticks are considered to be the second most common vector of human disease, after mosquitoes (8). Given this, the presence of a tick at the site of the rash implies that Suzanne's illness was likely caused by a tick-borne pathogen. Moreover, she is described as an avid hiker who was atAdmiral’s Cove recently, which increases her probability of catching a tick-borne illness (9).
Suzanne’s blood sample is taken to obtain her white blood cell (WBC) count as a higher-than-normal count is indicative of immune response activation and an increase in WBC production (9). A count of more than 11,000 leukocytes in a microliter of blood is considered a high WBC count, and Suzanne surpasses this with a count of 14,000, indicating leukocytosis and the presence of an infection (9). The rise in WBC count means that the bacterium is beginning to spread and has notified the immune response resulting in more immune cells being produced and migrating to the area of infection. To confirm that the infection is Lyme disease, the patient’s blood needs to be tested for antibodies by enzyme-linked immunosorbent assay (ELISA) and Western blot. (5,10).
For diagnosing infection of B. burgdorferi, causing Lyme disease, a sample of blood be taken from the patient and cultured of B. burgdorferi to determine the presence in the blood (11) However, this type of testing is not as reliable. It may result in false positives because is known to be able to inhibit the immune response and result in false-negative antibody results in 20-30% of patients (11). The more common method is to take a skin biopsy from the EM skin lesion and culture it in a BSK medium can determine the condition.
However, the two test-serological testings have also become a technique where the first enzyme-linked immunosorbent assay (ELISA) would be used and then a Western Blot (12). If the ELISA test comes back indeterminant or positive, it will be tested by Western blotting to confirm the diagnosis. This is due to serological testing being insensitive in the first few weeks of Lyme disease infection. An alone positive IgM results can be false positives if the illness has lasted longer than 4-8 weeks and there is no change in IgG levels and should not be used to confirm the diagnosis. If the patients present a distinguishable EM, serological testing may not be necessary (12).
References
1.Lyme disease [Internet]. Centers for Disease Control and Prevention. Centers for Disease Control and Prevention; 2022 [cited 2022Feb10]. Available from: https://www.cdc.gov/lyme/index.html#:~:text=Typical%20symptoms%20include%20fever%2C%20headache,of%20exposure%20to%20infected%20ticks
2. King LS. Signs and Symptoms. JAMA. 1968 Oct 28;206(5):1063–5.
3. Bockenstedt LK, Wormser GP. Unraveling Lyme Disease. Arthritis Rheumatol. 2014 Sep;66(9):2313–23.
4. Fever [Internet]. Johns Hopkins Medicine. [cited 2022Feb10]. Available from: https://www.hopkinsmedicine.org/health/conditions-and-diseases/fever#:~:text=Most%20healthcare%20providers%20consider%20a,higher-than-normal%20temperature.
5. Lyme disease [Internet]. Mayo Clinic. Mayo Foundation for Medical Education and Research; 2020 [cited 2022Feb10]. Available from: https://www.mayoclinic.org/diseases-conditions/lyme-disease/symptoms-causes/syc-20374651
6. CDC. Signs and symptoms of Lyme disease | CDC [Internet]. Centers for Disease Control and Prevention. 2021 [cited 2022 Feb 11]. Available from: https://www.cdc.gov/lyme/signs_symptoms/index.html
7. Skeff KM. Reassessing the HPI: The Chronology of Present Illness (CPI). J Gen Intern Med. 2014 Jan;29(1):13–5.
8. de la Fuente J, Estrada-Peña A, Venzal J, Kocan K, Sonenshine D. Overview: Ticks as vectors of pathogens that cause disease in humans and animals. Frontiers in bioscience : a journal and virtual library. 2008 Feb 1;13:6938–46.
9. Marques A. Chronic Lyme Disease: A Review. Infectious Disease Clinics of North America. 2008;22(2):341-360.
10. Crossland N, Alvarez X, Embers M. Late Disseminated Lyme Disease. The American Journal of Pathology. 2018;188(3):672-682.
11. Aguero-Rosenfeld ME, Pritt B, Adem PV, Wormser GP. Limitations and Confusing Aspects of Diagnostic Testing for Neurologic Lyme Disease in the United States. J Clin Microbiol. 2019 Jan 2;57(1):e01406-18.
12. Steere AC. MANDELL, DOUGLAS, AND BENNETT’S PRINCIPLES AND PRACTICE OF INFECTIOUS DISEASES. 9th edition. Philedelphia: Elsevier; 2019. 2911-2922.e2 p.
Question (ii): Fever and rash are two features in this case. How did the fever and the rash come about after exposure to the bacteria? In what way has the normal physiological functioning of this body system been disturbed by the infection (specifically looking at the physiological changes without detailing the bacterial mechanism of this disturbance as that is the basis of another question). Representing this diagrammatically is helpful to demonstrate understanding.
Rash
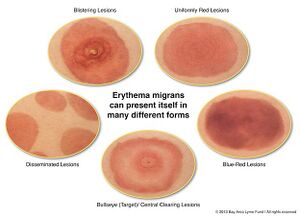
One of the most common clinical manifestations of Lyme disease is erythema migrans (EM), a skin rash that develops at the site of the tick bite after an incubation period of approximately 7-14 days (1). The rash usually resembles a bull's eye or a target; therefore, it is sometimes referred to as a "bull's eye" rash, as in Suzanne's case (1). EM typically starts off as a red macule which continues to expand, forming a large, annular, erythematous lesion (2). The skin lesion may range anywhere from 5-70cm in diameter (2). It usually contains a central clearing, or alternatively, the center of the lesion may be vesicular or necrotic (10). It is usually asymptomatic but can be pruritic or painful (2).
B. burgdorferi is introduced into dermal tissue by the bite of an infected tick, resulting in initiation of the host's innate immune response which leads to inflammation (6). The 5 main hallmarks of inflammation are redness, heat, swelling, pain, and loss of function; however, inflammation does not always lead to all 5 symptoms (3). In the case of EM, redness, heat, and swelling are observed, although some cases may involve pain as well (3).
As part of the innate immune response, immune cells release inflammatory mediators, such as bradykinin and histamine (3). Bradykinin and histamine are hormones that facilitate vasodilation, which allows more blood to reach the injured tissue, resulting in the redness and heat that is characteristic of EM (5). Increased blood flow is beneficial to the host because it increases the number of immune cells entering the infected area (3). Alongside vasodilation, bradykinin and histamine also increase blood vessel permeability, which makes it easier for immune cells to enter the infected region (3). The increased amount of fluid entering the inflamed tissue also leads to localized swelling (3). B. burgdorferi continues to replicate at the site of infection, eventually beginning to spread out at a speed of approximately 1-4 um per second which accounts for expansion of the redness of the rash (6). Additionally, the innate immune response is focused on the central portion of the infected region, which leads to faster clearance of B. burgdorferi in this area, eventually resulting in a central clearing (3).
Fever

Suzanne's fever was likely a side effect of severe inflammation (3). B. burgdorferi activates TLR1/2 homodimers by recognizing the triacylated lipid moiety on the cell surface lipopeptides, which initiates a signaling pathway of recruiting other immune cells to the site of infection, releasing pro-inflammatory cytokines: IL-6, IL-12, TNF-α and pro-IL-1β (13). The recognition of bacteria-induced a pro-inflammatory response Cytokines IL-1, IL-6 and TNF-α have been identified to be pyrogenic, meaning that they can induce higher body temperature (14).Fevers are usually triggered by the production of endogenous pyrogens, such as IL-1 and IL-6, from different macrophage-like cells which act on the anterior hypothalamus to increase the body's temperature (7). When the immune system is extremely active, a large amount of energy is needed to produce the required immune cells, as well as the appropriate antibodies (3). It has been hypothesized that the fever is the body's method of increasing the rate of metabolism to account for this increase in energy requirements (3).
Disruption of Normal Physiology
Initially, the tick bite will invade the skin barrier and release the bacteria under the epidermis, where the organism can migrate locally. Once the bacterium is ready to disseminate, it will initiate the EM rash seen to disrupt the extracellular matrix to gain entrance into the lymphatic system and blood to spread to organs or other skin sites (9). As well, illness may lead to reactive arthritis or atypical rheumatoid arthritis in adults (9).
The bacteria can spread to the tissues, organs, joints, heart, brain, and bladder, but it does not appear to circulate in the blood mainly but rather the lymphatic system, so blood tests may not be reliable (10). This bacterium is known to be able to spread to many body systems ranging from the central nervous system, heart and musculoskeletal.
Lyme disease can cross the lining of the blood-brain barrier and gain access into the CSF and inflame the meninges, resulting in meningitis, as well as affect the nerves in the face causing bell’s palsy. The bacteria can cross the BBB because of their ability to penetrate the lining with the use of plasminogen and break down the BBB to allow the invasion of the bacterium into the CNS. Then, these spirochete bacteria can burrow into the spinal cord and brain, which can cause the more severe symptoms of Lyme disease (11).
In addition, the bacteria can spread to the heart and interfere with the normal movement of the electrical signals in the heart that coordinate the heartbeat, causing a phenomenon known as “heart block”, leading to Lyme carditis (12).
References
- Patton SK, Phillips B. CE: Lyme Disease: Diagnosis, Treatment, and Prevention. AJN The American Journal of Nursing. 2018 Apr;118(4):38–45.
- Murray TS, Shapiro ED. Lyme Disease. Clin Lab Med. 2010 Mar;30(1):311–28.
- Marques AR. Lyme Disease: A Review. Curr Allergy Asthma Rep. 2010 Jan;10(1):13–20.
- Pike USNL of M 8600 R, MD B, Usa 20894. What is an inflammation? [Internet]. InformedHealth.org [Internet]. Institute for Quality and Efficiency in Health Care (IQWiG); 2018 [cited 2022 Feb 12]. Available from: https://www.ncbi.nlm.nih.gov/books/NBK279298/
- King LS. Signs and Symptoms. JAMA. 1968 Oct 28;206(5):1063–5.
- The bulls-eye rash of Lyme disease: Investigating the cutaneous host-pathogen dynamics of erythema migrans. [Internet]. ASM.org. [cited 2022 Feb 12]. Available from: http://www.yourdomain.com/index.php/general-science-blog/item/7250-going-skin-deep-investigating-the-cutaneous-host-pathogen-dynamics-of-erythema-migrans-the-bulls-eye-rash-of-lyme-disease
- Kluger MJ, Kozak W, Conn CA, Leon LR, Soszynski D. Role of Fever in Disease. Annals of the New York Academy of Sciences. 1998;856(1):224–33.
- Steere AC. MANDELL, DOUGLAS, AND BENNETT’S PRINCIPLES AND PRACTICE OF INFECTIOUS DISEASES. 9th edition. Philedelphia: Elsevier; 2019. 2911-2922.e2 p.
- M. Feder H, Abeles M, Bernstein M, Whitaker-Worth D, Grant-Kels JM. Diagnosis, treatment, and prognosis of erythema migrans and Lyme arthritis. Clin Dermatol. 2006 Nov 1;24(6):509–20.
- Borrelia Burgdorferi [Internet]. Bay Area Lyme Foundation. [cited 2022 Feb 11]. Available from: https://www.bayarealyme.org/about-lyme/what-causes-lyme-disease/borrelia-burgdorferi/
- Crystal J. Breaking Down Lyme Disease and the Blood-Brain Barrier [Internet]. Global Lyme Alliance. [cited 2022 Feb 11]. Available from: https://www.globallymealliance.org/blog/breaking-down-lyme-disease-and-the-blood-brain-barrier
- CDC. Lyme carditis | CDC [Internet]. Centers for Disease Control and Prevention. 2020 [cited 2022 Feb 11]. Available from:https://www.cdc.gov/lyme/treatment/lymecarditis.html
- Science I of M (US) C on LD and OT-BDTS of the. Pathogenesis [Internet]. Critical Needs and Gaps in Understanding Prevention, Amelioration, and Resolution of Lyme and Other Tick-Borne Diseases: The Short-Term and Long-Term Outcomes: Workshop Report. National Academies Press (US); 2011 [cited 2022 Feb 10]. Available from: https://www.ncbi.nlm.nih.gov/books/NBK57011/
- Conti B, Tabarean I, Andrei C, Bartfai T. Cytokines and fever. Front Biosci J Virtual Libr. 2004 May 1;9:1433–49.
Question (iii): What antibiotics might have been given (i.e., what are antibacterial treatments and how do these antibiotics work to help the body clear the organism)? Representing this diagrammatically is helpful to demonstrate understanding.
Lyme disease is an infectious disease caused by a gram-negative spirochete known as Borrelia burgdorferi1. Erythema migrans (EM) is a common objective manifestation of Lyme disease and it is present in approximately 90% of all cases1. EM is a skin lesion that begins as a small macule or papule centered around the site of the tick bite1. It grows at a rate of approximately 20cm2/day and later develops into a large erythematous patch1.
Clinicians usually prescribe oral or intravenous antibiotics in response to Lyme disease, and this method of treatment is most effective when administered in the early stages of disease progression2. Intravenous antibiotics, in particular, are usually involved in persistent or more severe and chronic Lyme disease; however, Suzanna is early on in the infection. Therefore, she will likely receive oral antibiotics. Commonly used antibiotics include orally administered amoxicillin, cefuroxime, and doxycycline as first-line agents for the treatment of EM3. Azithromycin may also be used in cases of intolerance to the aforementioned antibiotics4.
(i) Amoxicillin:
Amoxicillin is an antibiotic that was created by adding an extra amino group to another antibiotic known as penicillin to counter increasing resistance against penicillin5. It is usually prescribed as an alternative to doxycycline, as described below, in the case of children under eight or pregnant women6. Amoxicillin is administered orally three times a day for 14-21 days6. It is commonly used to treat infections caused by a wide variety of gram-positive bacteria, as well as a few gram-negative bacteria such as B. burgdorferi5.
The cell envelope of gram-positive bacteria is composed of a cytoplasmic membrane surrounded by a thick layer of peptidoglycan (PG), also known as murein, whereas gram-negative bacteria contain both an inner and an outer membrane with a thin layer of PG between them. Regardless, the cell envelope of both types of bacteria contains PG, which confers rigidness and mechanical stability7. Most importantly, however, PG prevents osmotic lysis of the bacterium7. As such, PG synthesis is a crucial component of the bacterial life cycle. PG consists of identical monomers joined together to form a linear polymer7. A single PG monomer is comprised of two amino sugars, N-acetylglucosamine (NAG) and N-acetylmuramic acid (NAM), linked in an alternating pattern through beta-(1,4)-glycosidic bonds7. Transglycosylase enzymes are responsible for joining these monomers together to form a linear polymer7. Additionally, the PG monomer contains a pentapeptide monomer coming off of the NAM. Pentapeptides from adjacent PG chains are joined together through peptide cross-links, resulting in the formation of a molecular chain link fence surrounding the bacterium7. Enzymes known as penicillin-binding proteins (PBPs) are bacterial transpeptidases that catalyze a transpeptidation reaction in the periplasm to facilitate peptide cross-linking7.
Amoxicillin belongs to a class of antibiotics known as beta-lactams, all of which feature a strained and reactive cyclic amide ring known as the beta-lactam ring8. Beta-lactams, such as amoxicillin, act by binding to PBPs and preventing their catalytic activity by inhibiting transpeptidation9. This, in turn, interrupts PG synthesis and leads to the activation of autolytic enzymes in the bacterial cell wall7,9. Ultimately, amoxicillin causes lysis of the cell wall and bacterial cell death9. This mechanism is known as bactericidal killing10.
(ii) Doxycycline:
Doxycycline is often the first-choice oral antibiotic used for the treatment of early and early disseminated Lyme disease, as well as late Lyme arthritis2,6,11. In early infection of B. burgdorferi, doxycycline is recommended for those eight years and older, excluding pregnant women12. After ten days of treatment with these antibiotics, it is seen that systemic symptoms of Lyme disease improved12. Doxycycline belongs to a class of antibiotics known as tetracycline antibiotics. First discovered in the 1940s, tetracyclines exhibit broad antimicrobial activity against both gram-positive and gram-negative bacteria, such as B. burgdorferi13. This class of antibiotics features a linear fused tetracyclic nucleus with various functional groups attached to it13.
Protein synthesis is essential to normal cell functioning. It can be broken down into two main parts: transcription and translation. Transcription refers to the production of messenger RNA, while translation involves using the mRNA as a template to produce proteins. Translation, in particular, can be divided into three main steps: initiation, elongation, and termination. Doxycycline acts as an antimicrobial agent by targeting elongation13. It accomplishes this by reversibly binding to the 30s ribosomal subunit of the bacteria, thus preventing the attachment of aminoacyl-tRNA to the acceptor (A) site of the ribosome13. As a result, the aminoacyl-tRNA is unable to transfer its amino acid to the growing polypeptide chain, resulting in inhibition of protein synthesis13. Doxycycline also binds to the 70S ribosome to further inhibit protein synthesis in the mitochondria14. Doxycycline also inhibits certain matric metalloproteases (MMPs), which are proteolytic enzymes, that are produced by inflammatory immune cells. Doxycycline hence has anti-inflammatory and anti-neoplastic activity14.
Tetracyclines moderate host-cell inflammatory responses and reduce microglial activation and proliferation, with inhibition of inducible nitric oxide synthase (iNOS) and IL-1β expression2. Tetracyclines inhibit the p38 MAPK and NFκB pathways, which play an important role in controlling the expression of pro-inflammatory mediators2. Moreover, these antibiotics may promote central nervous system (CNS) cell survival through inhibition of caspase-1 and caspase-3 activity2. Thus, tetracyclines may attenuate multiple processes involved in mediating inflammation and cell death. As a result, doxycyline significantly reduce the release of TNF-alpha, IL-6, and IL-8, IL-1, and IFN-alpha and beta2,11.
It should also be noted that tetracyclines, unlike beta-lactams, target molecules within the cytoplasm instead of the periplasm13. As a result, this class of antibiotics must have mechanisms to traverse the membranes of gram-positive or gram-negative bacteria. In gram-negative bacteria, such as B. burgdorferi, tetracyclines form coordination complexes with cations which allow them to enter the periplasm through the OmpF and OmpC porin channels. Next, the cationic metal is released, and the weakly lipophilic antibiotic diffuses through the lipid bilayer of the inner membrane13.
(iii) Cefuroxime axetil:
In the case of allergies to the two antibiotics mentioned above, cefuroxime axetil is used as a third-choice alternative for treating Lyme disease that should be taken orally twice a day for 14-21 days15. Cefuroxime is a second-generation cephalosporin that is able to target both gram-positive and gram-negative bacteria16. Similar to amoxicillin, cefuroxime is also a beta-lactam that disrupts PG synthesis by binding to PBPs, thus resulting in bacterial cell lysis16.
(iv) Azithromycin:
Azithromycin clears the body of infection by inhibiting bacterial protein synthesis17. It binds to 50S large ribosomal subunit and interferes with its assembly, as well as interferes with the growth of the nascent polypeptide chain. It binds at the polypeptide exit tunnel, which is close to the peptidyl transferase center on the 23S rRNA17. Azithromycin has a basic pH, which causes faster penetration of bacterial outer membranes and more effective entrance into it, hence increasing it antibacterial activity against gram-negative bacteria17.
References:
1. Dandache P, Nadelman RB. Erythema Migrans. Infectious Disease Clinics of North America. 2008 Jun 1;22(2):235–60.
2. Bernardino A, Kaushal D, Philipp M. The Antibiotics Doxycycline and Minocycline Inhibit the Inflammatory Responses to the Lyme Disease Spirochete Borrelia burgdorferi. The Journal of Infectious Diseases. 2009;199(9):1379-1388.
3. Cameron DJ, Johnson LB, Maloney EL. Evidence assessments and guideline recommendations in Lyme disease: the clinical management of known tick bites, erythema migrans rashes and persistent disease. Expert Review of Anti-infective Therapy. 2014 Sep 1;12(9):1103–35.
4. Caskey J, Hasenkampf N, Martin D, Chouljenko V, Subramanian R, Cheslock M et al. The Functional and Molecular Effects of Doxycycline Treatment on Borrelia burgdorferi Phenotype. Frontiers in Microbiology. 2019;10.
5. Akhavan BJ, Khanna NR, Vijhani P. Amoxicillin. In: StatPearls [Internet]. Treasure Island (FL): StatPearls Publishing; 2022 [cited 2022 Feb 10]. Available from: http://www.ncbi.nlm.nih.gov/books/NBK482250/
6. Taylor R, Simpson I. Review of Treatment Options for Lyme Borreliosis. Journal of Chemotherapy. 2005;17(sup2):3-16.
7. 2.3: The Peptidoglycan Cell Wall [Internet]. Biology LibreTexts. 2016 [cited 2022 Feb 10]. Available from: https://bio.libretexts.org/Bookshelves/Microbiology/Book%3A_Microbiology_(Kaiser)/Unit_1%3A_Introduction_to_Microbiology_and_Prokaryotic_Cell_Anatomy/2%3A_The_Prokaryotic_Cell_-_Bacteria/2.3%3A_The_Peptidoglycan_Cell_Wall
8. Fernandes R, Amador P, Prudêncio C. β-Lactams: chemical structure, mode of action and mechanisms of resistance. Reviews and Research in Medical Microbiology. 2013 Jan;24(1):7–17.
9. Akhavan, B. J., Khanna, N. R. & Vijhani, P. Amoxicillin. in StatPearls (StatPearls Publishing, 2022).
10. Marques AR. Lyme Disease: A Review. Curr Allergy Asthma Rep. 2010 Jan;10(1):13–20.
11. Shapiro ED. Borrelia burgdorferi (lyme disease). Pediatrics In Review. 2014;35(12):500–9.
12. Steere AC. MANDELL, DOUGLAS, AND BENNETT’S PRINCIPLES AND PRACTICE OF INFECTIOUS DISEASES. 9th edition. Philedelphia: Elsevier; 2019. 2911-2922.e2 p.
13. Chopra I, Roberts M. Tetracycline Antibiotics: Mode of Action, Applications, Molecular Biology, and Epidemiology of Bacterial Resistance. Microbiology and Molecular Biology Reviews [Internet]. 2001 Jun 1 [cited 2022 Feb 10]; Available from: https://journals.asm.org/doi/abs/10.1128/MMBR.65.2.232-260.2001
14. Holmes, N. E. & Charles, P. G. P. Safety and Efficacy Review of Doxycycline. Clinical Medicine. Therapeutics 1, CMT.S2035 (2009).
15. Maria Pacifici G. Clinical pharmacology of cefuroxime and cefuroxime axetil in infants and children. Clin Med Investig [Internet]. 2020 [cited 2022 Feb 11];5(3). Available from: https://www.oatext.com/clinical-pharmacology-of-cefuroxime-and-cefuroxime-axetil-in-infants-and-children.php#gsc.tab=0
16. Gold B, Rodriguez WJ. Cefuroxime: Mechanisms of Action, Antimicrobial Activity, Pharmacokinetics, Clinical Applications, Adverse Reactions and Therapeutic Indications. Pharmacotherapy: The Journal of Human Pharmacology and Drug Therapy. 1983;3(2):82–100.
17. Parnham, M. J. et al. Azithromycin: Mechanisms of action and their relevance for clinical applications. Pharmacology & Therapeutics 143, 225–245 (2014).
References (Images):
18. Amoxicillin. In: Wikipedia [Internet]. 2022 [cited 2022 Feb 18]. Available from: https://en.wikipedia.org/w/index.php?title=Amoxicillin&oldid=1069161989
19. Drusano GL. Antimicrobial pharmacodynamics: critical interactions of “bug and drug.” Nat Rev Microbiol. 2004 Apr;2(4):289–300.
20. Holmes NE, Charles PGP. Safety and Efficacy Review of Doxycycline. Clin Med Ther. 2009 Jan 1;1:CMT.S2035.
21. Graber EM. Treating acne with the tetracycline class of antibiotics: A review. Dermatological Reviews. 2021;2(6):321–30.
22. Cefuroxime axetil. In: Wikipedia [Internet]. 2021 [cited 2022 Feb 12]. Available from:https://en.wikipedia.org/w/index.php?title=Cefuroxime_axetil&oldid=1057597890
Question (iv): If Suzanne had not taken antibiotics immediately, what signs and symptoms could develop? What additional testing or monitoring could help with diagnosis in more advanced disease? Would this stage of the disease be treated differently?
Early Disseminated Disease
If she had not taken antibiotics during the early localized disease stage, Suzanne’s disease would have progressed to the next stage of clinical disease diagnosis. Early disseminated disease would occur from a few days to less than three months after initial infection(1). Typically within several weeks of localized erythema migrans, characteristic signs of early disseminated disease start to show, which are multiple secondary erythema migrans lesions, fever, arthralgias, lymphadenopathy and headache(1).
After a few weeks to less than 3 months, musculoskeletal, neurologic and/or cardiovascular manifestations can occur(1). Musculoskeletal symptoms are present in 60% of cases and include muscle or migratory joint pain with or without joint swelling(2). Neurologic signs include meningitis, radicular neuropathies, and paralysis of the facial cranial nerve. These are present in 15% of cases(2). The neuroborreliosis that may be observed, with or without aseptic meningitis, usually presents with cranial nerve involvement and occurs two to eight weeks after erythema migrans(3). If facial palsy is a sign in areas with Lyme disease endemic, health care professionals can suspect Lyme disease as the cause(4). Bell’s palsy, which is facial weakness and paralysis, can occur in 10-50% of children and adults in areas with Lyme-disease endemic(5). Analysis of cerebrospinal fluid (CSF) samples may be required if neuroborreliosis is suspected in Lyme-disease endemic areas(6). The abnormal CSF would have an elevated white blood cell count similar to that of viral meningitis(6). If the patient has Lyme meningitis, the CSF sample would have a higher mononuclear cell count and the patient would experience greater duration of headaches, possible cranial nerve involvement and a smaller chance of fever(7).
Cardiovascular abnormality signs are found in 8% of infection cases and may show temporary atrioventricular blocking to varying severities, and 30% of these patients may need temporary pacing, but complete heart blocks are rare(2). Usually a prolonged PR interval on electrocardiogram can present carditis(8). The Lyme carditis that occurs in 10% of patients is also rarely fatal with treatment being effective(9). However, in cases of Lyme carditis without signs of erythema migrans, serological blood testing is required to specify the disease-causing bacteria in order to administer correct therapy(10). The 2-tier serological test consists of ELISA or IFA as the first tier and a reflex Western immunoblot test as the second tier is used to check the antibody levels and confirm presence of disease(11). The same therapy as early localized disease for adults would be used to treat early disseminated disease, even if Bell’s palsy is a manifestation(5). However if CNS is involved, 100-200 mg of doxycycline for 28 days or IV administration of ceftriaxone (2 g) or Pen G (4 x106 units) for 14-28 days would be administered(1). For disease with carditis, the same treatment for CNS involvement is used but IV is recommended in cases if hospitalization is required(1). In infected children, 30 mg/kg of orally administered amoxicillin, penicillin or cefuroxime is provided for 14-21 days(1).
Late Disseminated Disease
Lyme disease may progress into late disseminated disease if the patient is untreated or inadequately treated(2). This can occur within weeks to months after the initial B. burgdorferi infection, and is seen in 60% of untreated cases(2). Clinical presentations of Lyme disease can vary based on the strain of B. burgdorferi. Arthritis is a common manifestation of late Lyme disease in North America, while neuroborreliosis is more common in Europe and presents as chronic encephalitis or radiculopathy that is called Bannwarth’s syndrome(12,13). If the patient has recently travelled to Europe and has signs of European Lyme disease, a serological test should be conducted to confirm the specificity of the disease(13).
Late Lyme arthritis is present in up to 60% of untreated Lyme disease cases. Swelling and intermittent pain of more than one joint may happen, with knees and hips being primarily affected(2,14). Most clinical manifestations of late Lyme arthritis occur within 2 years of initial infection(15). A serological test for diagnosis of Lyme arthritis is sensitive and specific, and can differentiate Lyme arthritis from septic arthritis, but the test takes time(16). Lyme arthritis involves the knee more than septic arthritis, but fever and local inflammation is less likely to accompany Lyme arthritis(17). Synovial inflammation (synovitis), occurs in up to 10% of patients six months after Lyme arthritis treatment(18). Arthrocentesis, which is the removal of synovial fluid from the joint capsule, can provide short-term symptomatic relief in some cases of synovitis, and also provide synovial fluid samples for Lyme polymerase chain reaction (PCR) tests(19). To determine if retreatment is needed for such patients, PCR test is used to detect B. burgdorferi DNA, but a positive test results after a primary treatment may not be significant(10). Previous research suggests that B. burgdorferi detected are not viable and questions if there is persistent infection in these patients(20). To treat late Lyme arthritis, administration of doxycycline (100 mg), amoxicillin (500 mg) or cefuroxime (500 mg) for 28 days if provided for cases without CNS involvement(1).
Manifestations of neuroborreliosis in late disseminated Lyme disease differ in North America and Europe, whereas in Europe Lyme disease is most commonly caused by Ixodes ricinus and Ixodes persulcatus(13,21) . Subacute encephalopathy with subtle cognitive changes in more common in North American cases, while chromic encephalomyelitis with spastic paraparesis and cognitive impairment is more frequently found in Europe(13). Treatment of late neuroborreliosis is similar for both North American and European neuroborreliosis(22). Infected adults are administered ceftriaxone (2 g) or Pen G (4 x106 units) for 14-28 days, while children are administered with varying pediatric dosing(1).
References
1. Hatchette T, Davis I, Johnston B. Lyme disease: clinical diagnosis and treatment. Can Commun Dis Rep. 2014 May 29;40(11):194–208.
2. Bratton RL, Whiteside JW, Hovan MJ, Engle RL, Edwards FD. Diagnosis and treatment of Lyme disease. Mayo Clinic Proceedings. 2008 May 1;83(5):566–72.
3. Logigian EL, Kaplan RF, Steere AC. Chronic Neurologic Manifestations of Lyme Disease. New England Journal of Medicine. 1990 Nov 22;323(21):1438–44.
4. Kuiper H, Devriese PP, de Jongh BM, Vos K, Dankert J. Absence of Lyme Borreliosis Among Patients With Presumed Bell’s Palsy. Archives of Neurology. 1992 Sep 1;49(9):940–3.
5. Clark JR, Carlson RD, Pachner AR, Sasaki CT, Steere AC. Facial paralysis in lyme disease. The Laryngoscope. 1985;95(11):1341–5.
6. Bremell D, Mattsson N, Wallin F, Henriksson J, Wall M, Blennow K, et al. Automated cerebrospinal fluid cell count — New reference ranges and evaluation of its clinical use in central nervous system infections. Clinical Biochemistry. 2014 Jan 1;47(1):25–30.
7. Eppes SC, Nelson DK, Lewis LL, Klein JD. Characterization of Lyme Meningitis and Comparison With Viral Meningitis in Children. Pediatrics. 1999 May 1;103(5):957–60.
8. Shapiro ED. Borrelia burgdorferi (Lyme Disease). Pediatr Rev. 2014 Dec 1;35(12):500–9.
9. Ray G, Schulz T, Daniels W, Daly ER, Andrew TA, Brown CM, et al. Three Sudden Cardiac Deaths Associated with Lyme Carditis — United States, November 2012–July 2013. MMWR Morb Mortal Wkly Rep. 2013 Dec 13;62(49):993–6.
10. Wormser GP, Dattwyler RJ, Shapiro ED, Halperin JJ, Steere AC, Klempner MS, et al. The Clinical Assessment, Treatment, and Prevention of Lyme Disease, Human Granulocytic Anaplasmosis, and Babesiosis: Clinical Practice Guidelines by the Infectious Diseases Society of America. Clinical Infectious Diseases. 2006 Nov 1;43(9):1089–134.
11. Moore A, Nelson C, Molins C, Mead P, Schriefer M. Current Guidelines, Common Clinical Pitfalls, and Future Directions for Laboratory Diagnosis of Lyme Disease, United States. Emerg Infect Dis. 2016 Jul;22(7):1169–77.
12. Pachner AR, Steiner I. Lyme neuroborreliosis: infection, immunity, and inflammation. The Lancet Neurology. 2007 Jun 1;6(6):544–52.
13. Hengge UR, Tannapfel A, Tyring SK, Erbel R, Arendt G, Ruzicka T. Lyme borreliosis. The Lancet Infectious Diseases. 2003 Aug 1;3(8):489–500.
14. The Clinical Evolution of Lyme Arthritis | Annals of Internal Medicine [Internet]. [cited 2022 Feb 13]. Available from: https://www.acpjournals.org/doi/abs/10.7326/0003-4819-107-5-725
15. Evaluation of Study Patients with Lyme Disease, 10–20-Year Follow-up | The Journal of Infectious Diseases | Oxford Academic [Internet]. [cited 2022 Feb 13]. Available from: https://academic.oup.com/jid/article/183/3/453/887055?login=true
16. Lindsay L, Bernat K, Dibernardo A. Laboratory diagnostics for Lyme disease. Can Commun Dis Rep. 2014 May 29;40(11):209–17.
17. Thompson A, Mannix R, Bachur R. Acute Pediatric Monoarticular Arthritis: Distinguishing Lyme Arthritis From Other Etiologies. Pediatrics. 2009 Mar 1;123(3):959–65.
18. Deanehan JK, Kimia AA, Tan Tanny SP, Milewski MD, Talusan PG, Smith BG, et al. Distinguishing Lyme From Septic Knee Monoarthritis in Lyme Disease–Endemic Areas. Pediatrics. 2013 Mar 1;131(3):e695–701.
19. Long KC, Cohn KA. Lyme Arthritis: An Update for Clinical Practice. Pediatric Emergency Care. 2018 Aug;34(8):588–91.
20. Li X, McHugh GA, Damle N, Sikand VK, Glickstein L, Steere AC. Burden and viability of Borrelia burgdorferi in skin and joints of patients with erythema migrans or lyme arthritis. Arthritis & Rheumatism. 2011;63(8):2238–47.
21. Marques AR, Strle F, Wormser GP. Comparison of Lyme Disease in the United States and Europe. Emerg Infect Dis. 2021 Aug;27(8):2017–24.
22. Oksi J, Nikoskelainen J, Hiekkanen H, Lauhio A, Peltomaa M, Pitkäranta A, et al. Duration of antibiotic treatment in disseminated Lyme borreliosis: a double-blind, randomized, placebo-controlled, multicenter clinical study. Eur J Clin Microbiol Infect Dis. 2007 Aug 1;26(8):571–81.
2. The Microbiology Laboratory
i) Including the stated bacterial cause, what are other bacterial pathogens associated with this type of exposure?
Lyme disease-causing bacteria
There are a variety of bacterial species that cause Lyme disease (LD), and they are all within the category of Borrelia burgdorferi sensu lato (Bbsl) (1). The Bbsl bacteria are spirochetes, meaning they share several molecular characteristics such as being spiral shaped gram-negative diderm bacteria (2). There are six genospecies of Bbsl that are human pathogenic: Borellia burgdorferi sensu stricto (Bbss), Borellia garinii, Borellia afzelii, Borellia bavariensis, Borrelia mayonii, and Borellia spielmani (1). Of these genospecies, only Bbss and B.mayonii are the causative agents of LD in North America (3, 4).
a) Borellia burgdorferi sensu stricto (Bbss)
In Canada, the main causative agent of LD is Bbss (5). The most common Bbss vector is a tick species known as Ixodes scapularis (black-legged tick), and less commonly Ixodes pacificus (western black-legged tick) (5). Peromyscus leucopus, the white-footed mouse, is the primary reservoir for I.scapularis growth, and consequently enables Bbss proliferation (5). Ticks are infected with Bbss when they feed on Bbss-infected animals (5). Ticks are capable of infecting humans with Bbss, but human infections stops the Bbss lifecycle, as they incapable of transmitting Bbss to other species or amongst themselves (dead-end host) (5). A tick bite results in the release of Bbss from the tick saliva into the host’s bloodstream (6). Following host entry, Bbss can disseminate into host tissues via adhesive proteins on the Bbss outer surface that are able to interact with host endothelial cell (EC) surface molecules (e.g., carbohydrates, proteins, and extracellular matrix elements) (7). Bbss is able to adjust the surface proteins it will display based on its surrounding environment (8). For instance, outer surface protein C (ospC) expression is induced in the midgut of the tick once the tick has attached to a host, and ospC allows for increased bacterial survival rates once in host bloodstream (8).
b) Borrelia mayonii
B.mayonii is a species of Bbsl that was identified in the upper midwestern United States in 2017 (9). The study sample size included only six patients, so requires further investigation to have more precise information on geographic distribution and whether it results in a difference in disease severity compared to Bbss (9). The authors noted that human infection with B.mayonii resulted in a diffuse rash, rather than the erythema migrans (EM) rash commonly seen in people infected with Bbss (9). As Suzzanne presented with an EM (bulls-eye) rash it is unlikely she was infected by B.mayonii (9).
Non-Lyme Disease-causing pathogens
Although this case leans heavily towards an LD diagnosis, there are at least two other bacterial-caused non-LD tick-borne diseases (TBDs) that have been identified in Canada (10). These non-LD TBDs are caused by the following bacterial pathogens: Anaplasma phagocytophilum and Borrelia miyamotoi (10).
a) Anaplasma phagocytophilum
A.phagocytophilum is spread to humans from tick bites and results in a disease know as Anaplasmosis. Common symptoms of Anaplasmosis include chills, fever, headache, and leukopenia, myalgia (all similar symptoms to LD), and in severe cases can lead to septic shock (11). Anaplasmosis does not result in EM, so it can be ruled out as a possible diagnosis for Suzanne (12).
b) Borrelia miyamotoi
B. miyamotoi is spread to humans from tick bites and results in a disease known as Borrelia miyamotoi disease (13). The disease manifests as recurrent spirochetemia (presence of spirochetes in blood circulation), relapsing fever, headache, chills, myalgia (14). It is uncommon for B. miyamotoi infection to cause an EM migrans rash common in LD. Polymerase chain reaction (PCR) is used to identify B. miyamotoi DNA to confirm Borrelia miyamotoi disease diagnosis (15).
Other infectious disease associated with rashes & fever:
Community clinics have been reported to misdiagnose 23–28% of EM rashes as cellulitis, shingles and ehrlichiosis (16). The most common causes of cellulitis is by Streptococcus and Staphylococcus bacterial infection. Bacteria enters and infects the tissue through breaks in the skin. Patients with cellulitis have similar symptoms as LD patients, such as a painful and red area of skin and fever (17). Shingles is the infection of an individual with the varicella zoster virus (VZV). This viral infection is characterized by a painful skin rash with blisters in a localized area and fever as well (18). Ehrlichiosis is diseases caused by the bacteria Ehrlichia chaffeensis, E. ewingii, or E. muris eauclairensis. These bacteria are spread to people primarily through the bite of infected ticks including the lone star tick (Amblyomma americanum) and the blacklegged tick (Ixodes scapularis) (19).People with ehrlichiosis will often have fever, chills, headache, muscle aches, and sometimes upset stomach (19).
References
1. Wolcott KA, Margos G, Fingerle V, Becker NS. Host association of Borrelia burgdorferi sensu lato: A review. Ticks Tick Borne Dis [Internet]. 2021;12(5):101766. Available from: http://dx.doi.org/10.1016/j.ttbdis.2021.101766
2. Bergström S, Normark J. Microbiological features distinguishing Lyme disease and relapsing fever spirochetes. Wien Klin Wochenschr [Internet]. 2018;130(15–16):484–90. Available from: http://dx.doi.org/10.1007/s00508-018-1368-2
3. Rudenko N, Golovchenko M, Grubhoffer L, Oliver JH Jr. Updates on Borrelia burgdorferi sensu lato complex with respect to public health. Ticks Tick Borne Dis [Internet]. 2011;2(3):123–8. Available from: http://dx.doi.org/10.1016/j.ttbdis.2011.04.002
4. Pritt BS, Mead PS, Johnson DKH, Neitzel DF, Respicio-Kingry LB, Davis JP, et al. Identification of a novel pathogenic Borrelia species causing Lyme borreliosis with unusually high spirochaetaemia: a descriptive study. Lancet Infect Dis [Internet]. 2016;16(5):556–64. Available from: http://dx.doi.org/10.1016/s1473-3099(15)00464-8
5. Bouchard C, Leonard E, Koffi JK, Pelcat Y, Peregrine A, Chilton N, et al. The increasing risk of Lyme disease in Canada. Can Vet J. 2015;56(7):693–9.
6. Grimm D, Tilly K, Byram R, Stewart PE, Krum JG, Bueschel DM, et al. Outer-surface protein C of the Lyme disease spirochete: a protein induced in ticks for infection of mammals. Proc Natl Acad Sci U S A [Internet]. 2004;101(9):3142–7. Available from: http://dx.doi.org/10.1073/pnas.0306845101
7. Caine JA, Coburn J. A short-term Borrelia burgdorferi infection model identifies tissue tropisms and bloodstream survival conferred by adhesion proteins. Infect Immun [Internet]. 2015;83(8):3184–94. Available from: http://dx.doi.org/10.1128/IAI.00349-15
8. Caine JA, Lin Y-P, Kessler JR, Sato H, Leong JM, Coburn J. Borrelia burgdorferiouter surface protein C (OspC) binds complement component C4b and confers bloodstream survival. Cell Microbiol [Internet]. 2017;19(12):e12786. Available from: http://dx.doi.org/10.1111/cmi.12786
9. Pritt BS, Respicio-Kingry LB, Sloan LM, Schriefer ME, Replogle AJ, Bjork J, et al. Borrelia mayonii sp. nov., a member of the Borrelia burgdorferi sensu lato complex, detected in patients and ticks in the upper midwestern United States. Int J Syst Evol Microbiol [Internet]. 2016;66(11):4878–80. Available from: http://dx.doi.org/10.1099/ijsem.0.001445
10. Public Health Agency of Canada. Tick-borne disease with climate and environmental changes - Canada.ca [Internet]. Canada.ca. 2019 [cited 2022 Feb 13]. Available from: https://www.canada.ca/en/public-health/services/reports-publications/canada-communicable-disease-report-ccdr/monthly-issue/2019-45/issue-4-april-4-2019/article-2-increased-risk-tick-borne-diseases-climate-change.html
11. Schotthoefer AM, Schrodi SJ, Meece JK, Fritsche TR, Shukla SK. Pro-inflammatory immune responses are associated with clinical signs and symptoms of human anaplasmosis. PLoS One [Internet]. 2017;12(6):e0179655. Available from: http://dx.doi.org/10.1371/journal.pone.0179655
12. Sanchez E, Vannier E, Wormser GP, Hu LT. Diagnosis, treatment, and prevention of Lyme disease, human granulocytic anaplasmosis, and babesiosis: A review. JAMA [Internet]. 2016;315(16):1767–77. Available from: http://dx.doi.org/10.1001/jama.2016.2884
13. Zinck CB, Lloyd VK. Borrelia burgdorferi and Borrelia miyamotoi in Atlantic Canadian wildlife. PLoS One [Internet]. 2022;17(1):e0262229. Available from: http://dx.doi.org/10.1371/journal.pone.0262229
14. Răileanu C, Tauchmann O, Vasić A, Wöhnke E, Silaghi C. Borrelia miyamotoi and Borrelia burgdorferi (sensu lato) identification and survey of tick-borne encephalitis virus in ticks from north-eastern Germany. Parasit Vectors [Internet]. 2020;13(1):106. Available from: http://dx.doi.org/10.1186/s13071-020-3969-7
15. Delaney SL, Murray LA, Aasen CE, Bennett CE, Brown E, Fallon BA. Borrelia miyamotoi serology in a clinical population with persistent symptoms and suspected tick-borne illness. Front Med (Lausanne) [Internet]. 2020;7:567350. Available from: http://dx.doi.org/10.3389/fmed.2020.567350
16. Aucott J, Morrison C, Munoz B, Rowe PC, Schwarzwalder A, West SK. Diagnostic challenges of early Lyme disease: lessons from a community case series. BMC Infectious Diseases. 2009 Dec;9(1):1-8.
17. Vary JC, O’Connor KM. Common dermatologic conditions. Medical Clinics. 2014 May 1;98(3):445-85.
18. Shafer WG, Hine MK, Levy BM, Rajendran R, Sivapathasundharam B. Shafer’s textbook of oral pathology. Diseases of the Skin. Rajendran R, editor. 2006;5:1103-7.
19. Rocky Mountain Spotted Fever (RMSF)". CDC. 15 November 2018. Retrieved 20 January 2019.
20. Borrelia burgdorferi (Lyme Disease)- An Overview [Internet]. Microbe Notes. [cited 2022 Feb 20]. Available from: https://microbenotes.com/borrelia-burgdorferi/
21. News Desk. Borrelia mayonii-like bacteria found in British Columbia ticks [Internet]. Outbreak News Today. 2016 [cited 2022 Feb 20]. Available from: http://outbreaknewstoday.com/borrelia-mayonii-like-bacteria-found-british-columbia-ticks-72075/
22. Mehlhorn H. Borrelia miyamotoi. In: Encyclopedia of Parasitology. Berlin, Heidelberg: Springer Berlin Heidelberg; 2016. p. 1–1.
ii) What samples could be taken for laboratory testing and how important is the Microbiology Laboratory in the diagnosis of this particular infectious disease?
Diagnosis of Lyme disease can be made based on clinical assessment alone, especially when erythema migrans is present and the patient was exposed to an environment in which B. burgdorferi-carrying ticks are present, as in Suzanne’s case (1). Erythema migrans presents as a bull’s eye-shaped rash, spanning up to 12 inches in diameter, and is one of the first symptoms of Lyme borreliosis, found in 70% to 80% of patients. Erythema migrans is a characteristic feature of Lyme disease, which otherwise has manifestations that overlap with common symptoms of many other illnesses, and therefore may be easily misdiagnosed. Patients will often present with erythema migrans before antibodies can develop, making the presence of erythema migrans a better indicator of early Lyme disease than laboratory tests (2). Thus, the presence of EM is considered sufficient to diagnose early LD in known endemic areas. However, because later infection stages of LD are less specific (3), physicians and experts should also take other factors into account, such as family history and existing health conditions, before taking patient samples to send to a microbiology laboratory to confirm or negate their theories. Lyme disease that does not present with erythema migrans may be difficult to diagnose, as antibodies take time to develop as part of the body’s immune response and so early Lyme disease may not be detected by antibody tests (4). As a result, antibody tests done during early Lyme disease will more likely than not yield false-negatives (2).
Nevertheless, laboratory test results in conjunction with clinical assessments are valuable, especially in the 20% to 30% of cases in which no erythema migrans is present, or when no B. burgdorferi-carrying tick populations have been established. In these instances, serology testing with a patient's blood samples is done, though cerebrospinal fluid (CSF) may also be used (6). Blood samples taken from an arm vein are the most common sample taken for LD diagnosis and can be used in a variety of tests (7). Blood should be collected in a sterile tube and whole blood can be stored at 4–8°C for up to 24 hours before the serum is separated. After separation, the serum should be carefully removed with a pipette to avoid extracting red blood cells, and transferred to a sterile vial (8). Ideally, serum specimens should be shipped to the laboratory as soon as possible. If the serum samples need to be kept for longer periods (more than 7 days), it should be frozen at −20°C or lower, and transported to the testing laboratory on frozen ice packs. Repeated freezing and thawing of serum samples for IgM testing should be avoided, as it may have detrimental effects on the stability of IgM antibodies (8). Currently, the Center for Disease Control and Prevention (CDC) recommends a two-tiered laboratory testing protocol for Lyme disease (LD) (3). Both tests are serology tests that work by measuring antibodies to Borrelia antigen in the patient’s blood sample (9). Both steps can be done using the same blood sample (3). In the case of a negative test result, further testing is not recommended, thus, only following either a positive or indeterminate result is the second step, involving an immunoglobulin (Ig) M or IgG immunoblot, performed. Lyme disease is only diagnosed when the first test is positive or equivocal and the second test is positive, although in some cases, the latter result may be equivocal as well (10).
Step one of standard two-tier testing involves an enzyme-linked immunosorbent assay (ELISA) test where laboratory technologists will look for the presence of anti-Borrelia burgdorferi antibodies (7). Subsequently, if anti-B. burgdorferi antibodies are detected, then step two, a Western blot can be run using the blood sample to make a confirmatory diagnosis (6). Negative results in either step of the standard two-tier testing process following recent infection is often where issues arise, as antibodies may take up to several weeks to develop to a testable, diagnostic level. As a result, early testing often produces false negatives for Lyme disease (12). This accounts for the relatively low accuracy rate for the Lyme disease serology test, as it is only correctly positive approximately 65% of the time, however, FDA-approved tests become more sensitive as the disease progresses and your body begins to mount an antibody response (13). There is also a high risk of a false positive serology test in instances where erythema migrans is not present and the probability of Lyme borreliosis is low, that is, when a patient has not traveled to a Lyme disease endemic area or does not live in a region that the ticks that spread Lyme disease habitat (14), and as such, serology testing is not recommended in these cases (15). In Canada, high-risk regions are located across the country but the vast majority of cases (95%) are reported in Ontario, Quebec, and Nova Scotia (16). Since Lyme disease only occurs in regions in which the environmental conditions can support the illness, testing is not required or recommended outside of these high-risk regions (2). Additionally, antibodies may stay in the blood for months and in some cases, years, even if the infection is gone (10). This means this test is not absolute and may result in false positive test results. This means not only may this testing not help or guide physicians in diagnosis, but it may even hinder diagnosis in some ambiguous cases.
Other laboratory tests include culturing plasma samples (obtained from blood samples) and staining them with acridine orange. This sample is then analyzed using fluorescent or dark-field microscopy for presence of B. burgdorferi (7). Also, lab technicians can perform polymerase chain reaction (PCR) on cultured plasma samples to detect B. burgdorferi DNA (7). However, PCR is more commonly used to test for B. burgdorferi in synovial fluid samples from LD patients with Lyme arthritis (7). When Lyme disease infection is suspected in the central nervous system, lumbar puncture (LP) is performed in which a needle is inserted into the spinal canal to collect cerebrospinal fluid (CSF) to diagnose Lyme neuroborreliosis (LNB) (17). When Lyme arthritis is suspected and the fluid in the joint is aspirated, the joint fluid may be tested for evidence of Lyme disease. Sometimes a skin biopsy sample is also taken to test for bacterial B. burgdorferi DNA in samples (18). However, the sensitivity of molecular testing is not sufficient for a negative result to rule out infection, especially in the cases of suspected neuroborreliosis (infection in CNS) (18). A group of researchers have also developed a system that used anti-OspaA monoclonal antibodies to detect B. burgdorferi antigen in urine samples of patients with an EM rash, and found 100% (24/24) patients had OspA protein in urine (19). However, this would only be used to confirm an LD diagnosis based on clinical presentation (19).
Laboratory tests are helpful to support diagnosis of LD from clinical presentations, but they are not required for diagnosis (7). Laboratory tests for LD are often unreliable as samples typically have low amounts of B. burgdorferi leading to false negative results (7). In Canada, EM rash with exposure to ticks is sufficient to make an LD diagnosis and microbiology laboratory testing is not recommended (20). If there is a tick exposure without EM rash and presence of other symptoms of LD (e.g., myalgia, headache, fever), then blood tests are recommended to confirm LD diagnosis (20). In addition to these laboratory tests, it is important for physicians to consider the signs and symptoms that a patient is exhibiting and expressing, the likelihood of exposure to ticks, and the possibility of similar illnesses. Thus, factors such as probability of disease and disease progression, and the patient’s medical and travel history must be taken into account when deciding whether or not to do serology testing (21). The importance of the microbiology lab in regards to a Lyme disease diagnosis is, therefore, most relevant in the case where there is moderate disease probability, persistent, unexplained symptoms, and the absence of erythema migrans.
References:
- Canadian Public Health Laboratory Network. The laboratory diagnosis of Lyme Borreliosis: Guidelines from the Canadian Public Health Laboratory Network. Canadian Journal of Infectious Diseases and Medical Microbiology. 2007Mar18;18(2):145–8.
- Shapiro ED. Borrelia burgdorferi (Lyme Disease). Pediatr Rev. 2014 Dec;35(12):500–9.
- Diagnosis and testing of Lyme disease | CDC [Internet]. Centers for Disease Control and Prevention. 2022 [cited 12 February 2022]. Available from: https://www.cdc.gov/lyme/diagnosistesting/index.html
- Lyme Disease Diagnostics Research | NIH: National Institute of Allergy and Infectious Diseases [Internet]. [cited 2022 Feb 9]. Available from: https://www.niaid.nih.gov/diseases-conditions/lyme-disease-diagnostics-research
- CNX OpenStax. Lyme Rash [Internet]. Wikimedia Commons. 2016 [cited 2022Feb19]. Available from: https://commons.wikimedia.org/wiki/File:OSC_Microbio_12_02_LymeRash.jpg
- Johnson RC. Leptospira [Internet]. Medical Microbiology. 4th edition. U.S. National Library of Medicine; 1996 [cited 2022Feb12]. Available from: https://www.ncbi.nlm.nih.gov/books/NBK8451/
- Marques AR. Laboratory diagnosis of Lyme disease: advances and challenges. Infect Dis Clin North Am [Internet]. 2015;29(2):295–307. Available from: http://dx.doi.org/10.1016/j.idc.2015.02.005
- Aucott J, Morrison C, Munoz B, Rowe PC, Schwarzwalder A, West SK. Diagnostic challenges of early Lyme disease: lessons from a community case series. BMC Infectious Diseases. 2009 Dec;9(1):1-8.
- Centers for Disease Control and Prevention (CDC. Recommendations for test performance and interpretation from the Second National Conference on Serologic Diagnosis of Lyme Disease. MMWR. Morbidity and mortality weekly report. 1995 Aug 11;44(31):590-1.
- Lyme disease diagnosis and testing. CDC. 2021. Available from: https://www.cdc.gov/lyme/signs_symptoms/index.html
- Spiritia. Serum Separation Tube [Internet]. Wikimedia Commons. 2019 [cited 2022Feb19]. Available from: https://commons.wikimedia.org/wiki/File:Serum-separation_tube.jpg
- Contributors WMDE. What is erythema migrans? what this rash means and how to treat it [Internet]. WebMD. WebMD; 2021 [cited 2022Feb13]. Available from: https://www.webmd.com/skin-problems-and-treatments/what-to-know-erythema-migrans#:~:text=Erythema%20migrans%20is%20a%20rash,be%20warm%20to%20the%20touch.
- Lyme Disease Frequently asked questions (FAQ) [Internet]. Centers for Disease Control and Prevention. Centers for Disease Control and Prevention; 2021 [cited 2022Feb13]. Available from: https://www.cdc.gov/lyme/faq/index.html#:~:text=Your%20immune%20system%20continues%20to,bacteria%20are%20no%20longer%20present.
- Suggested Reporting Language, Interpretation and Guidance Regarding Lyme Disease Serologic Test Results. :17.
- DePietropaolo DL, Powers JH, Gill JM, Foy A. Diagnosis of lyme disease [Internet]. American Family Physician. 2005 [cited 2022Feb13]. Available from: https://www.aafp.org/afp/2005/0715/p297.html
- Canada PHA of. Lyme disease surveillance in Canada: Preliminary annual report 2019 [Internet]. 2022 [cited 2022 Feb 10]. Available from: https://www.canada.ca/en/public-health/services/publications/diseases-conditions/lyme-disease-surveillance-report-2019.html
- Djukic M, Schmidt-Samoa C, Lange P, Spreer A, Neubieser K, Eiffert H, et al. Cerebrospinal fluid findings in adults with acute Lyme neuroborreliosis. J Neurol [Internet]. 2012;259(4):630–6. Available from: http://dx.doi.org/10.1007/s00415-011-6221-8
- Aguero-Rosenfeld ME, Wang G, Schwartz I, Wormser GP. Diagnosis of Lyme borreliosis. Clinical microbiology reviews. 2005 Jul;18(3):484-509.
- Magni R, Espina BH, Shah K, Lepene B, Mayuga C, Douglas TA, et al. Application of Nanotrap technology for high sensitivity measurement of urinary outer surface protein A carboxyl-terminus domain in early stage Lyme borreliosis. J Transl Med [Internet]. 2015;13(1):346. Available from: http://dx.doi.org/10.1186/s12967-015-0701-z
- Public Health Agency of Canada. Lyme disease: For health professionals [Internet]. Canada.ca. 2015 [cited 2022 Feb 13]. Available from: https://www.canada.ca/en/public-health/services/diseases/lyme-disease/health-professionals-lyme-disease.html
- CDC. Diagnosis and testing of Lyme disease | CDC [Internet]. Centers for Disease Control and Prevention. 2021 [cited 2022 Feb 8]. Available from: https://www.cdc.gov/lyme/diagnosistesting/index.html
iii) Explain the tests that can be performed on the samples in order to detect any of the potential bacterial pathogens associated with this type of exposure.
Current testing protocols:
The U.S. Centres for Disease Control (CDC) recommends a two-test system, where Lyme Disease can be diagnosed if both tests are positive, as no single serologic test is sufficiently sensitive or specific (1,2). These are currently two sets of tests cleared by the Food and Drug Administration (FDA) – STTT (standard two-tiered testing) and MTTT (modified two-tiered testing) (2). STTT consists of an enzyme-linked immunosorbent assay (ELISA) test, followed by a Western immunoblot assay if the first test is positive or equivocal (1). If the ELISA test is negative, then immunoblotting is not warranted (1). Recently, on July 29, 2019, the Food and Drug Administration (FDA) allows for an enzyme immunoassay (EIA) rather than western immunoblot assay as the second test in a Lyme disease diagnosis (3). This creates a new approach to antibody testing, MTTT. MTTT consists of two separate immunoassays, effectively replacing the western blot in STTT with EIA for the laboratory diagnosis of Lyme disease (3).
Algorithms for STTT (left) and MTTT (right) serotesting for detecting B. burdorferi infection.
Enzyme-linked immunosorbent assay (ELISA):
For an ELISA test, the patients blood sample will be sent to a laboratory and centrifuged, and the supernatant collected (serum sample) (4). There are four major types of ELISA tests, three of which can screen for the presence of antibodies in a sample (6). Direct ELISA and competitive ELISA are capable of detecting antibodies in samples, and indirect ELISA may detect both antibodies or antigens, while the target protein for sandwich ELISA is typically antigens. Of these, direct ELISA is the method of choice for diagnosing Lyme disease, as recommended by the CDC (7).
Western Blot:
A western blot detects anti-Borrelia antibody in a human serum sample(12). Only following a positive or indeterminate (sometimes referred to as an “unequivocal” result) is the second step of standard two-tier testing done (10;14). The same sample may be used to complete an IgM and IgG Western blot (also known as an immunoblot) to detect immunoglobulin specific to B. burgdorferi antigens (8; 10). Proteins from known Borrelia infected cells are separated and blotted on a membrane. Then, the serum to be tested is applied in the primary antibody incubation step. After that, free antibody is washed away and a secondary antibody is added which recognizes and binds to the primary antibody. The secondary antibody is visualized through various methods such as staining. The stained bands then indicate the proteins to which the patient's serum contains antibody (12).
In cases where testing is taking place within the first four weeks of disease onset, both IgM and IgG Western blots are done. After this four week period, only IgG immunoblots are recommended, as interpretation of IgM bands for a positive test is less reliable (8;10). The standardized criteria for positive IgM immunoblot requires at least two of three signature bands, while a minimum of five of ten signature bands is required for a positive IgG immunoblot (8). Western blots rely on protein separation by size based on molecular weight through gel electrophoresis, and then transfer to a solid-phase medium (15). The B. burgdorferi antigens are then incubated with preparations of patient serum. If antibodies (IgM and IgG) are present, they will bind to the antigens. A second solution, containing enzyme-linked antibodies to IgM and IgG is added. A positive test is indicated by a colour change induced by the activation of the enzyme-linked antibodies bound to the IgM- or IgG-antigen complex, which is visualized as a band (16).
Polymerase chain reaction (PCR) tests:
Another non-standard technique that can be used to detect presence of Borellia burgdorferi is a polymerase chain reaction (PCR) test (13). PCR can detect the presence of Borellia burgdorferi DNA from blood or CSF samples (13). If Borellia burgdorferi DNA is detected and clinical signs/symptoms of LD are present, this could serve as evidence for confirmation of LD diagnosis (13).
The benefit of this is that PCR can be used to identify an active B. burgdorferi infection which the ELISA test and western blot may not be able to identify due to the lengthy response time of the adaptive immune system (17). However, PCR testing is not commonly used to identify Lyme disease due to technical skill and equipment requirements, the lack of standards for PCR testing, and the risk of false-positives (17). Other challenges associated with PCR testing for B. burgdorferi include low concentrations of bacteria in blood and cerebrospinal fluid (CSF), the potential for laboratory contamination, and the invasive procedure required to acquire a test sample (18). For these reasons, identifying antibodies in the patient’s serum remains the primary means of confirming Lyme disease (18).
References:
- CDC. Diagnosis and testing of Lyme disease | CDC [Internet]. Centers for Disease Control and Prevention. 2021 [cited 2022 Feb 8]. Available from: https://www.cdc.gov/lyme/diagnosistesting/index.html
- Suggested Reporting Language, Interpretation and Guidance Regarding Lyme Disease Serologic Test Results. :17.
- Mead P, Petersen J, Hinckley A. Updated CDC Recommendation for Serologic Diagnosis of Lyme Disease. MMWR Morb Mortal Wkly Rep. 2019 Aug 16;68(32):703.
- Magnarelli LA, Ijdo JW, Padula SJ, Flavell RA, Fikrig E. Serologic diagnosis of Lyme borreliosis by using enzyme-linked immunosorbent assays with recombinant antigens. J Clin Microbiol [Internet]. 2000 [cited 2022 Feb 13];38(5):1735–9. Available from: https://www.ncbi.nlm.nih.gov/labs/pmc/articles/PMC86574/
- Crowther JR. ELISA: theory and practice. Springer Science & Business Media; 1995.
- Alhajj M. Enzyme linked immunosorbent assay [Internet]. StatPearls [Internet]. U.S. National Library of Medicine; 2021 [cited 2022Feb13]. Available from: https://www.ncbi.nlm.nih.gov/books/NBK555922/#:~:text=The%20primary%20antibod%20is%20added,detection%20of%20a%20color%20change.
- Canada PHAof. Modified two-tiered testing algorithm for Lyme disease serology: the Canadian context [Internet]. Modified two-tiered testing for Lyme disease serology: Canadian context, CCDR 46(5) - Canada.ca. / Gouvernement du Canada; 2020 [cited 2022Feb13]. Available from: https://www.canada.ca/en/public-health/services/reports-publications/canada-communicable-disease-report-ccdr/monthly-issue/2020-46/issue-5-may-7-2020/diagnostic-algorithm-lyme-disease-canada.html
- Marques AR. Laboratory diagnosis of lyme disease: Advances and challenges [Internet]. Infectious disease clinics of North America. U.S. National Library of Medicine; 2015 [cited 2022Feb13]. Available from: https://www.ncbi.nlm.nih.gov/pmc/articles/PMC4441761/
- Alhajj M. Enzyme linked immunosorbent assay [Internet]. StatPearls [Internet]. U.S. National Library of Medicine; 2021 [cited 2022Feb13]. Available from: https://www.ncbi.nlm.nih.gov/books/NBK555922/#:~:text=The%20primary%20antibod%20is%20added,detection%20of%20a%20color%20change.
- Johnson RC. Leptospira [Internet]. Medical Microbiology. 4th edition. U.S. National Library of Medicine; 1996 [cited 2022Feb12]. Available from: https://www.ncbi.nlm.nih.gov/books/NBK8451/
- Lyme Disease Frequently asked questions (FAQ) [Internet]. Centers for Disease Control and Prevention. Centers for Disease Control and Prevention; 2021 [cited 2022Feb13]. Available from: https://www.cdc.gov/lyme/faq/index.html#:~:text=Your%20immune%20system%20continues%20to,bacteria%20are%20no%20longer%20present.
- Sudha T, Lakshmi V, Teja VD. Western blot profile in HIV infection. Indian journal of dermatology venereology and leprology. 2006 Sep 1;72(5):357.
- Cerar T, Ogrinc K, Cimperman J, Lotric-Furlan S, Strle F, Ruzić-Sabljić E. Validation of cultivation and PCR methods for diagnosis of Lyme neuroborreliosis. J Clin Microbiol [Internet]. 2008;46(10):3375–9. Available from: http://dx.doi.org/10.1128/JCM.00410-08
- Diagnosis and testing [Internet]. Centers for Disease Control and Prevention. Centers for Disease Control and Prevention; 2021 [cited 2022Feb13]. Available from: https://www.cdc.gov/lyme/diagnosistesting/index.html
- Mahmood T, Yang P-C. Western blot: Technique, theory, and trouble shooting [Internet]. North American journal of medical sciences. Medknow Publications & Media Pvt Ltd; 2012 [cited 2022Feb13]. Available from: https://www.ncbi.nlm.nih.gov/pmc/articles/PMC3456489/
- Brenner C. Understanding the Western Blot [Internet]. Lyme Disease. 1996 [cited 2022Feb13]. Available from: https://www.lymedisease.org/assets/Understanding-Western-Blot-Lyme-disease-test.pdf
- Lyme Disease Test | HealthLink BC [Internet]. [cited 2022 Feb 9]. Available from:https://www.healthlinkbc.ca/tests-treatments-medications/medical-tests/lyme-disease-test
- Shapiro ED. Borrelia burgdorferi (Lyme Disease).Pediatr Rev. 2014 Dec;35(12):500–9.
iv) What are the expected results from these tests allowing for the identification of the bacteria named in this case. What are the test characteristics for these tests?
To identify B. burgdorferi, both step one and step two laboratory tests should yield positive or equivocal (1). If step one testing is negative, no further testing is commenced or required (1). These are the expected results for these tests in identifying B. burgdorferi as the causative agents for Lyme disease.
Initially, an enzyme-linked immunosorbent assay (ELISA), a diagnostic laboratory tool, is used to detect antibodies in the patient’s serum sample (2). It will test the binding of B. burgdorferi antigens to B. burgdorferi-specific antibodies in the patient’s serum sample (2). This binding forms an antigen-antibody complex, which is recognized by another enzyme type, such as alkaline phosphatase, horseradish peroxidase, or similar (3). This enzyme will generate a colour change via hydrolysis of a phosphate group by alkaline phosphatase or oxidation by horseradish peroxidase. This would confirm the presence of B. burgdorferi in the serum sample. However, this may result in a false positive test result because the proteins in B. burgdorferi used as recognizable antibodies may cross-react with similar proteins found in different bacterial pathogens (4). In other words, this means other bacterial pathogens that share similar proteins as B. burgdorferi, such as flagellar proteins, may yield a positive result as it detects the protein itself and not the source of the protein (4). One common cross-reactivity example is periodontal disease, which is often associated with an oral spirochete that has similar structures to B. burgdorferi spirochete, resulting in a false positive (4). Some other examples include the Epstein-Barr virus and human immunodeficiency virus (HIV), which are known to yield false positive results due to cross-reactivity (5). These false test results are unfortunately common, which is why the standards continue to be updated and why a two-tier testing system is used (5). A second follow-up test by Western blots is required to confirm B. burgdorferi-induced Lyme disease (4).
Western blots, an analytical laboratory technique that specifies proteins in a given mixture sample, which will separate the B. burgdorferi proteins and antigens by weight, measured in kilodaltons (kDa), and then exposed to the patient’s serum sample (4). These proteins and antigens will be bound by antibodies in the serum sample, and form corresponding bands on the immunoblot. These bands will indicate the specific types of proteins in the sample, and if they align with those in B. burgdorferi, experts can conclude the presence of B. burgdorferi, identifying them as the causative agent. However, there have been some discrepancies in analyzing and interpreting the immunoblot bands; thus, now, there are stricter standards on what constitutes a positive test result (6). For IgM test to be positive, there must be at least two of the following three bands in kDa: OspC (21-25), 39 (BmpA), and 41 (Fla) (7). For IgG test to be positive, there must be at least five of the following ten bands in kDa: 18, OspC (21-25), 28, 30, 39 (BmpA), 41 (Fla), 45, 58, 66, and 93 (7). With these updated standards on what constitutes a positive test result, it will help physicians diagnose Lyme disease by infection of B. burgdorferi clearer and with greater confidence.
Another thing to note about these test results is that it will help guide physicians on a treatment plan and diagnosis but are not a definitive indication. However, if a patient has been sick for more than 30 days and their IgM test result is positive, it should be treated as a false positive and disregarded (1). This is often why for Western blots, both IgM and IgG are recommended to be tested for. Furthermore, antibodies are known to persist in the serum for months or years even if the infection is gone (5). This means patients may test positive even though they have recovered, meaning it is a false positive and the underlying cause for their current signs and symptoms is something else.
Similarly, false negative tests are also an issue. If patients are tested too soon after exposure to B. burgdorferi, they may test negative because their immune response has not developed sufficiently and the antibodies are not in detectable amounts (8). This is because responding antibodies may take weeks to develop (1). Thus, if physicians suspect B. burgdorferi exposure and Lyme disease, they should re-conduct these tests with new samples 1-2 weeks later, as the adaptive immune response would likely have been properly triggered by then and antibodies will in detectable amounts (8).
These laboratory tests are powerful tools that assist experts in diagnosis, but physicians must look at the patient from a holistic perspective, taking into account their medical history, family history, signs and symptoms, time of exposure, the events leading to the first symptom, as well as changes as the disease develops.
References
- Lyme disease diagnosis and testing. CDC. 2021. Available from: https://www.cdc.gov/lyme/signs_symptoms/index.html
- Mead P, Petersen J, Hinckley A. 2019. Updated CDC Recommendation for Serologic Diagnosis of Lyme Disease. MMWR. Morbidity and mortality weekly report, 68(32), 703. https://doi.org/10.15585/mmwr.mm6832a4
- Alhajj M. Enzyme linked immunosorbent assay [Internet]. StatPearls [Internet]. U.S. National Library of Medicine; 2021 [cited 2022Feb13]. Available from: https://www.ncbi.nlm.nih.gov/books/NBK555922/
- Brenner C. Understanding the Western Blot [Internet]. Lyme Disease. 1996 [cited 2022 Feb 13]. Available from: https://www.lymedisease.org/assets/UnderstandingWestern-Blot-Lyme-disease-test.pdf
- Lyme Disease Test | HealthLink BC [Internet]. [cited 2022 Feb 9]. Available from: https://www.healthlinkbc.ca/tests-treatments-medications/medical-tests/lyme-disease-test
- Lantos PM, Auwaerter PG, Nelson CA. Lyme disease serology [Internet]. JAMA. U.S. National Library of Medicine; 2016 [cited 2022 Feb 13]. Available from: https://www.ncbi.nlm.nih.gov/pmc/articles/PMC5491346/
- Weinstein A. Laboratory testing for Lyme disease: time for a change? Clinical Infectious Diseases. 2008 Jul 15;47(2):196-7
- Suggested Reporting Language, Interpretation and Guidance Regarding Lyme Disease Serologic Test Results. :17
- John TM, Taege AJ. Appropriate laboratory testing in lyme disease. Cleveland Clinic Journal of Medicine [Internet]. 2019 Nov [cited 2022 Feb 20];86(11):751-9. Available from: https://www.ccjm.org/content/86/11/751 doi: 10.3949/ccjm.86a.19029
3. Bacterial Pathogenesis
Using the following pathogenic steps outline the pathogenesis of the bacteria named as being responsible for this infection.
i) Encounter: where does the organism normally reside, geographically and host wise, and what are the bacterial characteristics that leave it suited to these places of residence.
Geographic distribution of B. burgdorferi
The bulls-eye rash erythema migrans, one of the first symptoms of Lyme disease, is most commonly caused by the bacterium Borrelia burgdorferi, a spirochete bacterium that spreads to humans through infected arthropod vectors. Lyme disease is a multisystem, relapsing condition with common manifestations of rash and inflammation, and is currently the most prevalent vector-borne disease in North America, remaining as an important public health issue. On a global scale, Lyme disease is widespread in the northern temperate regions, which include regions of North America, central Europe, and forested areas of Asia.
In North America, this obligate parasite resides in a variety of small animals, though infection is typically asymptomatic unless in humans (1). Importantly, one of its natural reservoirs is deer ticks (also known as blacklegged ticks, or Ixodes scapularis) which can act as vectors for transmission and can be found in states throughout the northeastern and mid-Atlantic USA, such as Maine, New Hampshire, Massachusetts, Wisconsin, and Minnesota (2). In the US, the reported cases of Lyme disease vary between 25000-30000 per year (3). It is most prevalent in children ages 5 to 9 and adults 45 to 59, resulting in a bimodal distribution (3).
On the Pacific Coast of North America, B. burgdorferi resides within western blacklegged ticks (Ixodes pacificus), though it is less prevalent in the west due to these ticks being less capable of carrying B. burgdorferi (4,5). Ticks of the genus I. pacificus are mostly found on the western coast of the North American continent, and they primarily feed on lizards, which are not natural reservoirs for B. burgdorferi (6). Therefore, provinces such as B.C and Alberta have seen a relatively low incidence of Lyme disease. Across Europe and Asia, Lyme disease can be caused by other organisms in addition to B. burgdorferi, such as Borrelia afzelii, Borrelia garinii, and other related species. These organisms reside in the vectors Ixodes ricinus (the sheep tick) and Ixodes persulcatus (the taiga tick) in Europe and Asia respectively (7). Since Suzanne was travelling in Halifax (located in Eastern Canada, well within the normal range of I. scapularis and B. burgdorferi – see figure 1), we will be focusing on B. burgdorferi as the causative agent of Suzanne’s illness.
Being obligate parasites (evidenced by their limited genome size and lack of certain key metabolic pathways), the geographic distribution of B. burgdorferi is closely related to that of its primary host reservoir, Ixodes scapularis. These ticks can be found in regions which are favourable for its definitive host (the white-tailed deer), which are necessary for colonisation during the reproductive stage of I. scapularis (9). As larvae, the tick will feed on a variety of mammals and birds (most commonly the white-footed mouse), eventually transitioning to feeding on mostly large mammals such as the white-tailed deer (10) (See figure below describing tick life cycle). Although the presence of food sources is a critical factor in determining the geographic range of I. scapularis, other factors may affect its distribution, such as humidity (they are more commonly found in humid environments), vegetation (ticks prefer tall grass and forested areas), and season (although they can be found year-round, they are most likely to bite in the spring, according to the BC CDC) (5). The increase in B. burgdorferi infection seen in warmer months may be due to increased occurrences of questing ticks interacting with humans due to outdoor activities. Late spring/summer also aligns with the tick’s enzootic cycle. The I. scapularis life cycle usually lasts around 2 years, requiring 3 blood meals: one as a larvae, nymph, and adult (11) (See figure 2). Spring and summer seasons are when the I. scapularis mature from nymph to adult, most likely carrying B. burgdorferi when feeding on humans (11).
In eastern and central Canada, the incidence of Lyme disease in humans appears to have increased with climate change, perhaps indicating a shift in geographic localization of B. burgdorferi (5).
In Canada, the number of reported cases increased more than 6-fold between 2009 and 2015; this trend was observed mostly in eastern and central Canada. Data shown below is from the Canadian Notifiable Disease Surveillance System and Lyme Disease Enhanced Surveillance System show that southeastern Canada, specifically Nova Scotia, had the highest number of reported and acquired cases between 2009 and 2015 (13).
The most likely order of events in Suzanne’s case is as follows: prior to the transmission event, B. burgdorferi colonises the midgut of the tick. During feeding, it is the act of the midgut becoming engorged with blood which causes the pathogen to travel to the salivary glands, where it can be injected into the new host via saliva (7). A tick carrying B. burgdorferi can transmit the disease after filling itself with blood for about 24 hours (14). This corresponds with Suzanne’s history of infection where the tick’s head was buried in her calf overnight. She had also visited the harbor (specifically Halifax Harbor). Bodies of water are often popular spots for bird migration. These birds can carry and spread the tick vectors associated with B. burgdorferi (15).
Pathogen characteristics implicated in host colonization
Important characteristics of B. burgdorferi which aid in its pathogenic life cycle include its distinctive spiral morphology and the presence of periplasmic flagella, which aid in motility (16). These periplasmic flagella (also known as endoflagella) are attached to each pole of the bacterium, and work in coordination with each other as a ribbon-like structure to allow the organism to run, reverse, or flex/tumble during swimming (17). This mechanism for motility is highly regulated and plays an essential role in the invasion of host tissues in response to environmental signals (chemoattractants). Importantly, motility differs in zoonotic vectors vs. human hosts. In ticks, swimming behaviour is discontinuous and includes periods of non-motility (16). In mouse models of infection, B. burgdorferi has been observed in persistent motion in the dermis (18). Furthermore, the coordination of the flagella filaments allow the bacterium to move much faster than immune cells and navigate easily through viscous environments, such as those found in the connective tissues (19). As motility is a major virulence factor, experiments have shown that B. burgdorferi carrying mutations in the periplasmic flagella proteins had attenuated virulence and could not establish an infection in the host (20). As well, the endoflagella is encased within the periplasmic space, which is contained in-between a semi-rigid peptidoglycan helix and a flexible, outer membrane sheath with multiple layers. This arrangement enables B. burgdorferi’s survival and persistence in the host, as it can easily evade the immune system by hiding the highly antigenic flagella (14).
In fact, studies highlighting the importance of motility have shown that non-motile mutants of B. burgdorferi are unable to infect vertebrate hosts such as mice and humans (17,18). Other studies have shown that chemotaxis also contributes to the virulence of the pathogen (21). By deletion of the histidine kinase CheA2, which is essential for chemotaxis, Sze et al. demonstrated that inability to perform chemotaxis renders B. burgdorferi unable to establish infection in mice. Additional studies have investigated the role of adhesins which are expressed by B. burgdorferi during mammalian infection. It has been found that the pathogen attaches to the vascular endothelium when present in the bloodstream, which is believed to aid in its dissemination into other tissues. Strains which express very low levels of adhesins involved in tethering, dragging, and stationary events have been found to be avirulent – suggesting that adhesion is also involved in invasion and dissemination within host organisms (17). For example, a spirochetal protein binds αIIb β3, a platelet-specific integrin, and a glycosaminoglycan binding protein binds heparan sulphate on endothelial cells (22). Another protein BBK32 on the bacterium binds to fibronectin, an extracellular matrix protein expressed ubiquitously.
These mechanisms which enable B. burgdorferi to invade and colonise hosts are highly regulated, allowing them to be controlled when establishing infection in the tick. Some studies have found that although motile behaviour presents differently in tick vs. human/mammal hosts, motility is nonetheless essential for long-term survival of B. burgdorferi within the tick. This is evidenced by the fact that non-motile mutants of the pathogen are unable to replicate within infected ticks and are even quickly cleared by the tick immune response (18). It is hypothesised that motility of the pathogen promotes adhesion to epithelial cells in the tick midgut (interaction between the bacterial outer surface protein OspA and the corresponding tick receptor TROSPA) (23). Pal et al. have also shown that a decrease in TROSPA expression following feeding is linked to B. burgdorferi egressing from the tick, supporting the role of adhesion in the establishment of B. burgdorferi infection and transmission.
Other characteristics which are used by B. burgdorferi to colonise the tick midgut include those which allow it to evade tick immune defences and endocytic digestion by epithelial cells in the gut (12). It does this by interacting with and modulating the gut environment, along with preferentially expressing certain genes to adapt to changes in pH or temperature (24). In addition to facilitating adherence to epithelial cells, the outer membrane proteins OspA and OspC also play a role in protection against innate immune responses in the tick. They bind plasminogen and catalyse conversion to plasmin, which can negatively regulate the complement system and dampen the innate immune response, preventing clearance of the pathogen (12). OspA is also believed to be involved in shielding B. burgdorferi from antibodies in the tick gut. Other bacterium surface proteins like DbpA and DbpB bind Decorin, a proteoglycan found on the surface of collagen, and P66 binds integrins. Various host cell integrins and extracellular matrix components are involved, such as the collagen fibrils in the extracellular matrix of the heart, nervous system, and joints. These interactions allow B. burgdorferi to persist, and mutations in these proteins reduce infectivity as the bacterium loses protection from the host extracellular matrix (1).
In addition to the negative regulation of immune responses, B. burgdorferi can also evade the complement system by altering the expression of surface proteins at different stages of infection (25). For example, the VlsE lipoprotein encoded by a linear plasmid known as lp28-1 undergoes extensive antigenic alterations during infection, while another immunogenic surface protein OspC is downregulated during infection. Additional regulatory systems exist which allow B. burgdorferi to be able to scavenge for essential nutrients in the gut environment by modulating metabolic flexibility to be able to utilise different nutrients when available (12).
In summary, despite its limited genome size, B. burgdorferi makes use of several regulatory systems, motile behaviour, and membrane-expressed factors in order to successfully reside within a wide range of hosts.
References:
- Tilly K, Rosa PA, Stewart PE. Biology of Infection with Borrelia burgdorferi. Infect Dis Clin North Am [Internet]. 2008 Jun [cited 2022 Feb 10];22(2):217–34. Available from: https://www.ncbi.nlm.nih.gov/pmc/articles/PMC2440571/
- Centers for Disease Control and Prevention. Lyme Disease Maps: Most Recent Year [Internet]. Centers for Disease Control and Prevention; 2021 [cited 2022 Feb 8]. Available from: https://www.cdc.gov/lyme/datasurveillance/maps-recent.html
- Tatum R, Pearson-Shaver AL. Borrelia Burgdorferi. In: StatPearls [Internet]. Treasure Island (FL): StatPearls Publishing; 2022 [cited 2022 Feb 7]. Available from: http://www.ncbi.nlm.nih.gov/books/NBK532894/
- CDC. Transmission of Lyme disease | CDC [Internet]. Centers for Disease Control and Prevention. 2020 [cited 2022 Feb 10]. Available from: https://www.cdc.gov/lyme/transmission/index.html
- Lyme Disease [Internet]. [cited 2022 Feb 10]. Available from: http://www.bccdc.ca/health-info/diseases-conditions/lyme-disease-borrelia-burgdorferi-infection
- Eppes S, Goldstein ND. Lyme Disease (Borrelia burgdorferi). Nelson Textbook of Pediatrics. Chapter 249, 1604-1609.e1
- Shapiro ED. Borrelia burgdorferi (Lyme Disease). Pediatr Rev [Internet]. 2014 Dec [cited 2022 Feb 10];35(12):500–9. Available from: https://www.ncbi.nlm.nih.gov/pmc/articles/PMC5029759/
- Provincial Health Authority of Canada. Lyme disease: Monitoring [Internet]. 2015 [cited 2022 Feb 11]. Available from: https://www.canada.ca/en/public-health/services/diseases/lyme-disease/surveillance-lyme-disease.html
- Lubelczyk CB, Elias SP, Rand PW, Holman MS, Lacombe EH, Smith RP Jr. Habitat Associations of Ixodes scapularis (Acari: Ixodidae) in Maine. Environ Entomol [Internet]. 2004 Aug 1 [cited 2022 Feb 10];33(4):900–6. Available from: https://doi.org/10.1603/0046-225X-33.4.900
- Thevanayagam S. Ixodes scapularis (black legged tick) [Internet]. Animal Diversity Web. [cited 2022 Feb 10]. Available from: https://animaldiversity.org/accounts/Ixodes_scapularis/
- Mead PS. Epidemiology of Lyme disease. Infect Dis Clin North Am. 2015 Jun;29(2):187–210.
- Kurokawa C, Lynn GE, Pedra JHF, Pal U, Narasimhan S, Fikrig E. Interactions between Borrelia burgdorferi and ticks. Nat Rev Microbiol [Internet]. 2020 Oct [cited 2022 Feb 11];18(10):587–600. Available from: https://www.nature.com/articles/s41579-020-0400-5
- Gasmi S, Ogden NH, Lindsay LR, Burns S, Fleming S, Badcock J, Hanan S, Gaulin C, et al. Surveillance for Lyme Disease in Canada: 2009 – 2015 [Internet]. The Public Health Agency of Canada; 2017 Oct [cited 2022 Feb 7];43(1). Available from: https://www.canada.ca/en/public-health/services/reports-publications/canada-communicable-disease-report-ccdr/monthly-issue/2017-43/ccdr-volume-43-10-october-5-2017/surveillance-surveillance-lyme-disease-canada-2009-2015.html
- Todar K. Todar’s Online Textbook of Bacteriology. University of Wisconsin Madison Dept. of Bacteriology. Bacterial Pathogens and Diseases of Humans. 2008. Available from: http://textbookofbacteriology.net/
- Talbot B, Slatculescu A, Thickstun CR, Koffi JK, Leighton PA, McKay R, et al. Landscape determinants of density of blacklegged ticks, vectors of Lyme disease, at the northern edge of their distribution in Canada. Sci Rep. 2019 Nov 13;9(1):16652.
- Hyde JA. Borrelia burgdorferi Keeps Moving and Carries on: A Review of Borrelial Dissemination and Invasion. Front Immunol [Internet]. 2017 [cited 2022 Feb 10];8. Available from: https://www.frontiersin.org/article/10.3389/fimmu.2017.00114
- Motelab MA, Liu J, Wooten RM. Spirochetal motility and chemotaxis in the natural enzootic cycle and development of Lyme disease. Curr Opin Microbiol [Internet]. 2015 Dec [cited 2022 Feb 10];28:106–13. Available from: https://www.ncbi.nlm.nih.gov/pmc/articles/PMC4688064/
- Sultan SZ, Sekar P, Zhao X, Manne A, Liu J, Wooten RM, et al. Motor Rotation Is Essential for the Formation of the Periplasmic Flagellar Ribbon, Cellular Morphology, and Borrelia burgdorferi Persistence within Ixodes scapularis Tick and Murine Hosts. Infect Immun [Internet]. 2015 Feb 17 [cited 2022 Feb 10]; Available from: https://journals.asm.org/doi/abs/10.1128/IAI.03097-14
- Charon NW, Goldstein SF. Genetics of motility and chemotaxis of a fascinating group of bacteria: The spirochetes. Annu Rev Genet. 2002 Jun. Available from: https://pubmed.ncbi.nlm.nih.gov/12429686/
- Dombrowski C, Kan W, Motaleb MA, Charon NW, Goldstein RE, Wolgemuth CW. The Elastic Basis for the Shape of Borrelia burgdorferi. Biophys J. 2009 Jun;96(11): 4409–4417. Available from: https://pubmed.ncbi.nlm.nih.gov/19486665/
- Sze CW, Zhang K, Kariu T, Pal U, Li C. Borrelia burgdorferi Needs Chemotaxis To Establish Infection in Mammals and To Accomplish Its Enzootic Cycle. Infect Immun [Internet]. 2012 Jul [cited 2022 Feb 11];80(7):2485–92. Available from: https://www.ncbi.nlm.nih.gov/pmc/articles/PMC3416460/
- Zemel L, Bockenstedt LK. Lyme Disease. Textbook of Pediatric Rheumatology. 45, 602-612.e5 2020.
- Pal U, Li X, Wang T, Montgomery RR, Ramamoorthi N, Desilva AM, et al. TROSPA, an Ixodes scapularis receptor for Borrelia burgdorferi. Cell. 2004 Nov 12;119(4):457–68.
- Kenedy MR, Lenhart TR, Akins DR. The role of Borrelia burgdorferi outer surface proteins. FEMS Immunol Med Microbiol [Internet]. 2012 Oct 1 [cited 2022 Feb 12];66(1):1–19. Available from: https://doi.org/10.1111/j.1574-695X.2012.00980.x
- Lin YP, Diuk-Wasser MA, Stevenson B, Kraiczy P. Complement Evasion Contributes to Lyme Borreliae–Host Associations. Trends Parasitol.2020 May;36:7:34-645. Available from: https://doi.org/10.1016/j.pt.2020.04.011
ii) Entry: what facilitates the entry of the bacteria into the human host? What are the molecular, cellular and/or physiological factors at play in the initial entry/adherence step from the point of view of the organism and the host.
Acquisition of B. burgdorferi by the tick
As previously established, the blacklegged tick is the primary vector for Lyme disease-causing B. burgdorferi (1). Since most tick-borne infections are transmitted by the bite of this tick, I. scapularis, it is important to understand the interactions between the bacteria and the tick before moving onto the human host. The I. scapularis life cycle spans between 2 to 4 years and includes egg, larval, nymphal, and adult stages, as demonstrated in Figure 2 of the previous section. Tick-borne pathogens such as B. burgdorferi are usually acquired during the nymphal or larval feed and then transmitted to hosts by adults (1). Immature ticks are more generalist feeders, feeding on small and medium-sized mammals, birds, and reptiles (1). The primary host of adult ticks is usually the white-tailed deer, although adult female ticks also feed on humans and domestic animals which can then acquire B. burgdorferi but are not as important to further perpetuate infection (1).
As shown in Figure 1, The I. scapularis tick takes up B. burgdorferi along with bloodmeal through feeding from an infected host. SALP25D salivary protein is injected into the host skin in order to dampen inflammation at the feeding site, which enhances acquisition of the bacteria by the tick. A chemoattractant, SALP12, functions to increase the quantity of spirochetes which enter the tick. The spirochetes that are ingested in the bloodmeal then adhere to the gut of the tick and remain there until the next feeding. The B. burgdorferi expresses outer surface proteins OspA and OspB which protect it from harmful components such as antibodies or complement within the host’s blood, and then enabling them to adhere and persist in the tick gut.
Changes in protein expression are key in the migration of the bacteria from the tick midgut to the human. Within the tick midgut, the Borrelia’s outer surface protein A (OspA) binds to the tick receptor for OspA, known as TROPSA. This allows the bacterium to retain residency within the tick midgut during the tick’s non-feeding periods (Hovius, van Dam & Fikrig, 2007). When bloodmeal content increases in the midgut, the tick will downregulate OspA and upregulate OspC, another surface protein. OspC is able to bind to Salp15, a tick salivary protein. Binding to Salp15 has two primary benefits for the tick; firstly, it allows the tick to localize from the gut to the salivary glands, and secondly, binding to Salp15 has immunosuppressive effects on the tick’s immune system, which allows for increased bacterial reproduction and by extension, increased bacterial shedding into the Tick’s saliva (16). OspC knockout mutant spirochetes are completely unable to invade the tick salivary gland, and by extension, are unable to be transmitted to the host (16).
Entry into Human Host
I. Factors at play from the pathogen’s perspective
In most cases, a tick carrying B. burgdorferi must attach to the host and feed for at least 24 hours before the pathogen can be transmitted (17), and this aligns with our case where Suzanne has left the tick on her calf overnight.
As the tick bites, B. burgdorferi is deposited onto the skin along with the tick saliva (see figure below). This process is enhanced by the immunomodulatory properties of the tick saliva, which promotes feeding and impairs host hemostasis and immune response (18). Even if the tick is removed from the host, the bacterium is already deposited and can cause an infection. The strain of Borrelia affects how likely it is that it will enter the host bloodstream.
The tick midgut microenvironment is vastly different from the metabolic, chemical, and physical conditions within mammalian tissue. To survive in the host, B. burgdorferi must be able to rapidly acclimate to these conditions. To facilitate its survival, and eventual dissemination through the bloodstream, B. burgdorferi is capable of changing glycoprotein and protease expression on its cell surface (15). Once exposed to bloodmeal B. burgdorferi expresses outer surface proteins that selectively interact with host endothelial cells, platelets, chondrocytes, and extracellular matrix via specific interactions with integrins, glycosaminoglycans, fibronectin, and collagen. While the mechanisms by which B. burgdorferi invades and colonises the host are poorly understood, the capacity of B. burgdorferi to bind a variety of cells and extracellular matrix proteins gives an indication that they may express adhesive molecules. A summary of these outer surface proteins, the host receptor they bind to, and their function is provided below:
· P66: Integrin αIIbβ3: Attachment of spirochetes to platelets is mediated by the platelet-specific integrin αIIbβ3 (4), also known as GPIIb/IIIa. Integrins are heterodimeric receptors which are divalent-cation-dependent and mediate a variety of cell-cell and cell-extracellular matrix interactions (4). The specificity of integrins is determined by the combination of alpha and beta polypeptide chains, and the amino acid sequence Arg-Gly-Asp (RGD) is present in several mammalian ligands (4). P66 primarily binds to fibrinogen, and is largely responsible for the formation of platelet aggregates at sites of endothelial damage. αIIbβ3 exists in an inactive form on resting platelets. Upon endothelial damage, however, αIIbβ3 changes conformation in response to platelet activation. This change in conformation allows P66 to bind to the integrin. Endothelial damage caused by the tick bite, therefore, indirectly contributes to B. burgdorferi adhesion within the host by activating platelets. Activation of the platelets allows for the attachment of spirochetes to platelets that are attached to the vessel wall at sites of endothelial damage, such as the tick bite site (3). Therefore, P66: Integrin αIIbβ3 interactions are key for bacterial adhesion to the host at the site of entry. It has been shown that Borrelia strains that did not bind platelets were noninfectious (5), which indicates that the adhesion of B. burgdorferi to platelets via the αIIbβ3 platelet-specific, activation-dependent fibrinogen receptor is important for the pathogenesis of Lyme disease (5).
· BBK32: Fibronectin: Fibronectin is a complex glycoprotein, present both in plasma and in extracellular matrix, which contains distinct domains that interact with heparin, collagen, gelatin, integrins and other plasma and tissue components (4). BBK32 is a surface lipoprotein which is upregulated by B. burgdorferi in ticks after the initiation of the blood meal. It is produced in the mammalian host has been shown to adhere to host fibronectin (6). The ability of BBK32 to bind to Fibronectin, which will be present in tissue and clotting sites at the site of the tick bite, can act as a potential mechanism of bacteria:host adhesion at the site of bacterial entry.
· DbpA/B: Decorin: In human tissues, B. burgdorferi appears to be associated with connective tissue in general and with collagen fibers in particular (1). The bacteria binds to Decorin, a collagen-binding proteoglycan that is produced as a component of the connective tissue in multiple human tissues (8). There have been several studies which have shown that DbpA/B: Decorin interactions appear to play a key role in initial bacterial adhesion and dissemination. Firstly, DbpA and DbpB are not expressed by B. burgdorferi during infection of the tick; its expression is upregulated exclusively during mammalian and murine infection (9). Secondly, B. burgdorferi disseminates somewhat less efficiently in Decorin-deficient mice than in wild-type controls (2). In these mutant mice, B. burgdorferi survives less well in the skin, a tissue which contains relatively high-levels of Decorin transcript and is amongst the initial tissues which B. burgdorferi interacts with upon host entry.
· Bgp: Heparan-SO4/Dermatan-SO4 (GAG): Glycosaminoglycans (GAGs) are a diverse group of long linear polysaccharides consisting of repeating disaccharide units. B. burgdorferi is capable of binding to non-Decorin GAG chains produced by a wide variety of mammalian cells (4). The type of GAGs that mediate cell attachment by the bacterium varies with mammalian cell type. For example, heparan sulphate plays the predominant role in binding of the spirochete to cultured endothelial cells, whereas dermatan sulphate and heparan sulphate contribute to bacterial attachment to cultured glial cells (4). Bgp (Borrelia GAG-binding Protein) is one of the GAG-binding proteins that has been identified. GAGs are ubiquitously expressed across human tissues; the ability of B. burgdorferi to interact and adhere to GAGs is thought to play an instrumental role in bacterial adhesion and eventually dissemination.
On top of that, the pathogen can also suppress immune responses to a certain extent. For instance, proteins such as Erps and CRASP help B. burgdorferi inhibit immune responses by binding to host complement-mediated killing proteins, while another protein OspC inhibits the activation CD4 T cells and the classical pathway. Together, these properties allow B. burgdorferi to enter the host successfully and establish infection.
The ‘bull’s eye’ appearance of erythema migrans is therefore a result of two inflammatory responses: the central part to the salivary proteins from the tick, and the outer ring(s) that correspond to the dissemination of B. burgdorferi from the initial site of infection.
In addition to the virulence factors mentioned above, the physical features of B. burgdorferi also play an important role in its adherence to and movement along the host vasculature. One end of B. burgdorferi usually has adhesins that are more adherent, and that end is always pointing in the direction opposite to blood flow instead of following a passive arrangement to resist the shear stress of blood flow (7).
Another physiological feature that aids B. burgdorferi’s survival in response to changes in environmental conditions between the tick and mammalian host is pleomorphism. It has been suggested that when transmitted by the tick, B. burgdorferi transforms from a spirochete to round body forms with lower metabolic activity. This transformation affects B. burgdorferi’s ability in evading the immune system and responding to antibiotic treatment (11).
II. Factors at play from the host’s perspective
From the host perspective, the overall health state of the individual plays a role in containing and responding to infections. Baseline defense mechanisms such as mucosal secretions of antibacterial factors and rapid mucosal epithelial cells replacement are involved in responding to the initial inoculation and entry of pathogen.
One important piece of information from Suzanne’s case is that she visited multiple breweries and ate lobster following the tick bite. Undercooked seafood may contain a wide range of bacteria and parasites, and acute exposure to high levels of alcohol can compromise the immune system defense mechanisms, making individuals more susceptible to potential infections and less capable to respond to existing infections (13).
On a physiological basis, fever is an effective way for the mammalian host to counteract the initial infection. Using whole-genome arrays, researchers have identified temperature-induced changes in B. burgdorferi’s gene expressions. For example, genes that encode dihydroxyacetone phosphate which enhances the rate of energy production are repressed at higher temperatures (12). This in turn would hinder B. burgdorferi’s motility and infectivity.
On a molecular basis, mutations in components involved in the innate immune response can affect the host’s ability to respond to the pathogen. For example, deficiency in the TLR2 pattern recognition receptors is associated with significantly elevated bacterial loads in skin tissues at the initial site of infection; a similar observation is found in those lacking MyD88, a component essential for signaling pathways involving various pattern recognition receptors (16). However, the difference in bacterial load diminishes in various body organs during the later stages of infection.
References
- Barthold, S. W., de Souza, M. S., Janotka, J. L., Smith, A. L., & Persing, D. H. (1993). Chronic Lyme borreliosis in the laboratory mouse. The American journal of pathology, 143(3), 959–971.
- Brown, E. L., Wooten, R. M., Johnson, B. J. B., Iozzo, R. V., Smith, A., Dolan, M. C., Guo, B. P., Weis, J. J., & Höök, M. (2001). Resistance to lyme disease in Decorin-deficient mice. Journal of Clinical Investigation, 107(7), 845–852. https://doi.org/10.1172/jci11692
- Coburn J, Barthold S, Leong J. Diverse Lyme disease spirochetes bind integrin alpha IIb beta 3 on human platelets. Infection and Immunity. 1994;62(12):5559-5567.
- Coburn, J., Fischer, J. R., & Leong, J. M. (2005). Solving a sticky problem: New genetic approaches to host cell adhesion by the lyme disease spirochete. Molecular Microbiology, 57(5), 1182–1195. https://doi.org/10.1111/j.1365-2958.2005.04759.x
- Coburn J, Magoun L, Bodary S, Leong J. Integrins α v β 3 and α 5 β 1 Mediate Attachment of Lyme Disease Spirochetes to Human Cells. Infection and Immunity. 1998;66(5):1946-1952.
- Dombrowski C, et al. (2009). The Elastic Basis for the Shape of Borrelia burgdorferi. Biophysics Journal. 96(11): 4409–4417
- Fikrig, E., Feng, W., Barthold, S. W., Telford, S. R., & Flavell, R. A. (2000). Arthropod- and host-specific borrelia burgdorferi bbk32 expression and the inhibition of spirochete transmission. The Journal of Immunology, 164(10), 5344–5351. https://doi.org/10.4049/jimmunol.164.10.5344
- Guo, B. P., Norris, S. J., Rosenberg, L. C., & Höök, M. (1995). Adherence of borrelia burgdorferi to the proteoglycan decorin. Infection and Immunity, 63(9), 3467–3472. https://doi.org/10.1128/iai.63.9.3467-3472.1995
- Hagman, K. E., Lahdenne, P., Popova, T. G., Porcella, S. F., Akins, D. R., Radolf, J. D., & Norgard, M. V. (1998). Decorin-binding protein of borrelia burgdorferi is encoded within a two-gene operon and is protective in the murine model of Lyme Borreliosis. Infection and Immunity, 66(6), 2674–2683. https://doi.org/10.1128/iai.66.6.2674-2683.1998
- Kurokawa C, Lynn G, Pedra J, Pal U, Narasimhan S, Fikrig E. Interactions between Borrelia burgdorferi and ticks. Nature Reviews Microbiology. 2020;18(10):587-600.
- Merilainen L, et al. 2015. Morphological and biochemical features of Borrelia burgdorferi pleomorphic forms. Microbiology Society. 161:3
- Ojaimi C, et al. 2003. Profiling of Temperature-Induced Changes in Borrelia burgdorferi Gene Expression by Using Whole Genome Array. Infection and Immunity. 71(4): 1689–1705
- Pavia CS, et al. 2002. Impaired Bactericidal Activity and Host Resistance to Listeria Monocytogenes and Borrelia Burgdorferi in Rats Administered an Acute Oral Regimen of Ethanol. Clinical and Diagnostic Laboratory Immunology, American Society for Microbiology
- Petnicki-Ocwieja T, Kern A. Mechanisms of Borrelia burgdorferi internalization and intracellular innate immune signaling. Frontiers in Cellular and Infection Microbiology. 2014;4.
- Probert W, Kim J, Höök M, Johnson B. Mapping the Ligand-Binding Region of Borrelia burgdorferi Fibronectin-Binding Protein BBK32. Infection and Immunity. 2001;69(6):4129-4133.
- Tilly, K., Rosa, P. A., & Stewart, P. E. (2008). Biology of infection with borrelia burgdorferi. Infectious Disease Clinics of North America, 22(2), 217–234. https://doi.org/10.1016/j.idc.2007.12.013
- Todar K. 2008. Todar, 2008's Online Textbook of Bacteriology. University of WisconsinMadison Dept. of Bacteriology. Bacterial Pathogens and Diseases of Humans.
- Zemel L; Bockenstedt LK. 2021. Lyme Disease. Textbook of Pediatric Rheumatology.45, 602-612.e51.
- Kurokawa C, Lynn G, Pedra J, Pal U, Narasimhan S, Fikrig E. Interactions between Borrelia burgdorferi and ticks. Nature Reviews Microbiology. 2020;18(10):587-600.
iii) Multiplication and Spread: does the organism remain extracellular or do they enter into cells and what are the molecular and cellular determinants of these events. Do the bacteria remain at the entry site or do they spread beyond the initial site i.e. are there secondary sites of infection and why do the bacteria hone in on these particular secondary sites.
Internalisation
B. burgdorferi is typically referred to as an extracellular pathogen which does not possess an intracellular niche (1). However, it has also been observed as being able to invade human endothelial cells as far back as 1991, when Ma et al. suggested that the persistence of B. burgdorferi in tissues during late-stage Lyme disease (despite prominent anti-pathogen immune responses) implied the pathogen’s ability to ‘hide’ from the immune response – either in an immunologically protected tissue or within the host cells (2). Using an in vitro model, they showed that B. burgdorferi is able to invade human umbilical vein endothelial cells in an actin-dependent manner. More recently, several groups have shown that B. burgdorferi is able to be internalised by various cell types, including endothelial cells, fibroblasts, neuronal, and neuroglial cells (3).
Additional evidence of B. burgdorferi internalisation into host cells is demonstrated by the signaling pathways that are triggered in response to the pathogen. In particular, B. burgdorferi has been shown to trigger signaling pathways downstream of both Toll-like receptors (TLRs) and NOD-like receptors (NLRs, which are localised in intracellular compartments) to activate the innate immune response (1). Although specific NLR engagement in response to B. burgdorferi remains to be studied, it is well-established that TLR2 interacts with cell surface B. burgdorferi lipoproteins (such as OspA) to contribute significantly to innate response signaling (4).
The internalisation of B. burgdorferi begins with adhesion to the host cell and is followed by engulfment. Adhesion (explained in detail in the previous section) typically involves bacterial adhesins interacting with integrins on the surface of the host cell (for example, integrin αvβ3 binds the p66 protein of B. burgdorferi). In particular, Hawley et al. have demonstrated that integrin αMβ2 mediates bacterial attachment while working in conjunction with CD14 (a GPI anchored receptor associated with TLR2) to mediate engulfment of the bacteria into the host cell (5). Once bound, integrin αMβ2 contributes to the recruitment of Toll-interleukin 1 receptor (TIR) domain-containing adapter protein (TIRAP) to membranes rich in phosphatidylinositol 4,5-biphosphate (PIP2). The interaction between TIRAP and PIP2 recruits the MyD88 signaling adapter to initiate cell surface signaling (6). MyD88 functions at the plasma membrane to trigger signaling cascades and eventually lead to the activation of MAP kinases and NF-kB, which further activate pro-inflammatory cytokines such as IL-6, IL-12, TNF-a, and pro-IL1β (see Figure 1 below) (1).
Internalisation of B. burgdorferi into host cells is not as well-characterised as the mechanism of adhesion. Since discovering that engulfment occurs by coiling (a process where the host cell surface rolls the attached bacterium into a single fold of the plasma membrane) in 1992, the precise cellular signaling pathways which regulate this event have remained unknown (7). More recently, it was shown that the formation of f-actin rich structures occurs during phagocytosis, and that the pathways involved in actin polymerization and bacterial internalisation are modulated by Cdc42 and Rac1 (small GTPases) in addition to PI3K signaling (8). However, there is still a significant gap in our knowledge of how cell surface molecules like integrins and CD14 function together to regulate the complicated process of signaling and internalisation.
Dissemination
Once inside the host, B. burgdorferi disseminates, with the signs of this disseminated infection presenting in the musculoskeletal, cardiovascular, and nervous systems (10). Borrelia burgdorferi can disseminate by binding strongly to both the extracellular matrix and the cells of connective tissue (11). Dissemination From the site of the tick bite within the mammalian host to secondary infection sites requires dramatic adaptation of the pathogen in response to environmental changes and evading host innate immune responses (12). After infection, which can usually range from days to weeks, B. burgdorferi progresses onto a disseminated stage when the bacteria travel away from the initial site of the tick bite through the bloodstream and through the lymphatic system to invade, infect and colonise various tissues of the human host, such as the heart, synovial fluid of joints, and the nervous system, including both the peripheral nervous system (PNS) and the central nervous system (CNS) (12). It is believed this shift in pathogenic behaviour occurs in part due to signaling via the MyD88 pathway, which causes the bacterium to adapt and circumvent the localised immune response (12). Infected individuals can develop secondary erythema migrans at distal locations on the skin from the original site of infection (12). Late infection stages, often referred to as the third stage of disease, develop months to years after exposure to B. burgdorferi, and infected individuals can experience different manifestations including neuroborreliosis, Lyme carditis, and arthritis (12).
Dissemination of B. burgdorferi and subsequent invasion of mammalian tissues in the subsequent stages of the disease is an important step for pathogenesis and immune evasion of the bacteria. Invasion of B. burgdorferi by entering into the tissue through transmigration or extravasation results in the colonisation of secondary infection sites that become the foci of disease. B. burgdorferi has the ability to preferentially escape into the heart, the joint tissue or to cross the blood-brain barrier (BBB), stimulating an inflammatory immune response to cause carditis, arthritis, and neuroborreliosis (12). The endothelial surface of the BBB has a unique organisation and increased level of protection in comparison to other endothelial tissues targeted for invasion by B. burgdorferi.
Hematogenous Vascular Interactions
B. burgdorferi dissemination in mammals can occur through two routes: the hematogenous and the non-hematogenous routes, such as through the lymphatic system or direct spread through the tissues (12). The most prevalent form of dissemination is by far the hematogeneous routes, which will be the focus of this section.
Three ribosomal spacer type (RST) genotypes have been defined for B. burgdorferi as RST1, RST2, and RST3 in descending order of dissemination capabilities (1). At least 16 different ospC genotypes vary in the ability to disseminate (12). More human infections have been associated with ospC types A, B, I, H, and K (12).
After initiating a localised dermal infection, B. burgdorferi begins to disseminate throughout the mammalian host via the hematogenous route. Studies have defined B. burgdorferi dissemination interactions as transient tethering-type associations, dragging interactions, and stationary adhesion (12). Transient tethering-type interactions occur briefly when B. burgdorferi slows down relative to the speed of the bloodstream in a way that ties cells to the endothelium at a single point or tip before detaching (12). Tethering reduces the dissociation rates of B. burgdorferi from the endothelium, which in turn stabilises interactions. An additional type of short-term interaction is dragging, which is distinguished from tethering by B. burgdorferi interacting with the endothelium along the length of the cell and being dragged with blood flow across the cell surface (12). Stationary adhesion is the final stage of vascular interaction prior to transmigration. During this type of interaction, the B. burgdorferi cell is at a complete stop and no longer travelling in the direction of the bloodstream (12). It is worth noting that fibronectin-binding protein (BBK32) is involved in all three types of interactions. Stationary interaction through BBK32 may be through direct interaction with the endothelial surface or through a fibronectin bridge to a host integrin (12). P66 also interacts with integrins and is important for transmigration of B. burgdorferi.
Since the bacteria can have strong interactions with integrins, dissemination to diverse tissues may also be promoted via the binding of the bacteria to platelets (10). Spirochetes are concentrated at regions of endothelial damage as a result, which are the areas in which activated platelets would be the most common. Adhesion to platelets may also contribute to transmission of B. burgdorferi, as tissue damage surrounding the tick attachment site may result in platelet activation (10). The integrin αIIbβ3 (glycoprotein IIb-IIIa) and αvβ3 (vitronectin receptor), which may account for colonisation of the vasculature (14), is a critical receptor in thrombosis and hemostasis, and also mediates the binding of B. burgdorferi to human platelets which facilitates dissemination of the bacteria to secondary sites after the initial infection site (13). Binding to platelets at the infection site in the skin provides a foothold for the establishment of infection, an area where the bacteria can replicate while staying protected from the host defence system by a surrounding thrombus (13).
Additionally, the pathogen expresses proteins (such as DbpA, which promotes transient tethering with epithelial cells (12)) that bind the proteoglycan decorin, which is known to associate with collagen (14). Importantly, collagen is distributed widely throughout the ECM of the nervous system, joints, and the heart. Finally, a glycosaminoglycan-binding (GAG-binding) protein expressed by B. burgdorferi interacts and mediates binding to GAG side chains of heparin and dermatan sulfate (found on neuronal cells) (14). These factors may contribute to preferential dissemination of B. burgdorferi into specific tissues.
Is there intracellular persistence?
It has been reported that human synovial cells, responsible for producing synovial fluid components in the joints, are a target for intracellular persistence of B. burgdorferi after the bacteria pass through endothelial barriers (15). Initial internalisation of the bacteria into endothelial cells is mediated by an expressed endothelial receptor, but not adhesion molecules (16). B. burgdorferi has also been reported to enter macrophages rapidly and result in degradation and apparent intracellular persistence, which gives an indication of one of the possible reservoirs for chronic and recurrent Lyme disease in humans (16). However, it is more common to hear of B. burgdorferi persisting extracellularly in ligaments and collagen tissue of Lyme disease patients (15), and there is a higher detection of bacterial DNA in these tissue samples (15). As well as this, there are also reports of extracellular matrix localization of B. burgdorferi in tissues other than joints which leads to the conclusion that the bacteria generally seems to reside extracellularly (16). Any intracellular persistence seems to be a rare event, but further studies are recommended in order to verify this.
References:
- Petnicki-Ocwieja T, Kern A. Mechanisms of Borrelia burgdorferi internalization and intracellular innate immune signaling. Front Cell Infect Microbiol [Internet]. 2014 Dec 15 [cited 2022 Feb 12];4:175. Available from: https://www.ncbi.nlm.nih.gov/pmc/articles/PMC4266086/
- Ma Y, Sturrock A, Weis JJ. Intracellular localization of Borrelia burgdorferi within human endothelial cells. Infect Immun [Internet]. 1991 Feb [cited 2022 Feb 12];59(2):671–8. Available from: https://www.ncbi.nlm.nih.gov/pmc/articles/PMC257809/
- Wu J, Weening EH, Faske JB, Höök M, Skare JT. Invasion of Eukaryotic Cells by Borrelia burgdorferi Requires β1 Integrins and Src Kinase Activity. Infect Immun [Internet]. 2010 Dec 20 [cited 2022 Feb 12]; Available from: https://journals.asm.org/doi/abs/10.1128/IAI.01188-10
- Hirschfeld M, Kirschning CJ, Schwandner R, Wesche H, Weis JH, Wooten RM, et al. Cutting edge: inflammatory signaling by Borrelia burgdorferi lipoproteins is mediated by toll-like receptor 2. J Immunol Baltim Md 1950. 1999 Sep 1;163(5):2382–6.
- Hawley KL, Olson CM, Iglesias-Pedraz JM, Navasa N, Cervantes JL, Caimano MJ, et al. CD14 cooperates with complement receptor 3 to mediate MyD88-independent phagocytosis of Borrelia burgdorferi. Proc Natl Acad Sci U S A. 2012 Jan 24;109(4):1228–32.
- Kagan JC, Medzhitov R. Phosphoinositide-mediated adaptor recruitment controls Toll-like receptor signaling. Cell. 2006 Jun 2; 125(5):943-55. [Internet]. [cited 2022 Feb 12]. Available from: https://pubmed.ncbi.nlm.nih.gov/16751103/
- Rittig MG, Krause A, Häupl T, Schaible UE, Modolell M, Kramer MD, et al. Coiling phagocytosis is the preferential phagocytic mechanism for Borrelia burgdorferi. Infect Immun. 1992 Oct;60(10):4205–12.
- Shin OS, Miller LS, Modlin RL, Akira S, Uematsu S, Hu LT. Downstream signals for MyD88-mediated phagocytosis of Borrelia burgdorferi can be initiated by TRIF and are dependent on PI3K. J Immunol Baltim Md 1950. 2009 Jul 1;183(1):491–8.
- Coburn J, Leong J, Chaconas G. Illuminating the roles of the Borrelia burgdorferi adhesins. Trends Microbiol. 2013 Aug;21(8):372-9. Available from: https://www.ncbi.nlm.nih.gov/pmc/articles/PMC3773214/
- Coburn J, Barthold S, Leong J. Diverse Lyme disease spirochetes bind integrin alpha IIb beta 3 on human platelets. Infect Immun. 1994;62(12):5559-5567.
- Zambrano, MC, Beklemisheva, AA., Bryksin, AV, Newman, SA, Cabello, FC. Borrelia burgdorferi binds to, invades, and colonizes native type I collagen lattices. Infect Immun. 2004 Jun;72(6), 3138–3146. Available from: https://doi.org/10.1128/IAI.72.6.3138-3146.2004
- Hyde, JA. Borrelia burgdorferi keeps moving and carries on: A review of Borrelial dissemination and invasion. Front Immunol. 2017 Feb;8:114. Available from: https://www.ncbi.nlm.nih.gov/pmc/articles/PMC5318424/
- Coburn J, Leong J, Erban J. Integrin alpha IIb beta 3 mediates binding of the Lyme disease agent Borrelia burgdorferi to human platelets. Proc Natl Acad Sci U S A. 1993;90(15):7059-7063.
- Steere AC, Coburn J, Glickstein L. The emergence of Lyme disease. J Clin Invest. 2004 Apr;113(8):1093–101.
- Kumar Singh S, Josef Girschick H. Molecular survival strategies of the Lyme disease spirochete Borrelia burgdorferi. Lancet Infect Dis. 2004;4(9):575-583.
- Dorward D. Interactions Between Mouse Lymphocytes And Borrelia Burgdorferi, The Infectious Agent Of Lyme Disease. Microsc Microanal. 1999;5(S2):1242-1243.
iv) Bacterial Damage: do the bacteria cause any direct damage to the host (or is the damage fully attributable to the host response, as indicated below) and, if so, what is the nature of the bacterial damage. Can it be linked to any of the signs and symptoms in this case?
B. burgdorferi can cause direct damage to the host in terms of degrading host extracellular matrix (ECM) proteins, as well as indirect damage to the host through inflammation in various tissues of the host. B. burgdorferi HtrA protease (BbHtrA), a surface-exposed serine protease expressed during human disease, degrades fibronectin and numerous proteoglycans found in skin, joints and neural tissues (2). The human HtrA orthologue, HtrA1, plays a large role in extracellular matrix (ECM) remodeling with many substrates including aggrecan, decorin, growth factors and fibronectin (2). Fibronectin fragments, and those of other ECM molecules such as biglycan and decorin, can stimulate inflammatory responses by engaging cell surface receptors (2). BbHtrA degradation of fibronectin can release known pro-inflammatory fibronectin fragments, which may amplify the inflammatory responses triggered by the presence of the bacteria and the initial establishment of the infections.
Borreliella species, which B. burgdorferi belongs to, do not produce potent exotoxins or invasion-enhancing enzymes but cause infection by migrating through the skin and other tissues, disseminating in the blood to other organs, adhering to host cells, and evading innate and adaptive immunity (4). Although Lyme disease spirochetes do not produce lipid A-containing lipopolysaccharide, they do produce lipoproteins that are ligands for toll-like receptors on mononuclear blood cells and other cells, primarily white blood cells that have phagocytic activities (4). Binding to these receptors can lead to the release of pro-inflammatory cytokines with effects qualitatively similar to responses to endotoxin (4).
Erythema migrans
Erythema migrans, a form of early cutaneous Lyme disease, develop at tick bite sites and appears as ring-like erythema (3). Standard histopathologic descriptions include a superficial and deep perivascular lymphocytic infiltrate in which plasma cells are identified at the periphery of the lesion and eosinophils in the center (3). In other words, erythema migrans indicate an intradermal battle between the bacteria and the host immune system. The primary lesion of erythema migrans appears 3–30 days after the tick bite in 50%–83% of cases of Lyme disease and is associated with systemic symptoms of fever, fatigue, malaise and arthralgias (3).
As Lyme disease progresses onto the late stage, it will spread throughout the body and affect multiple organs and tissues. Some examples are the central nervous system (CNS), muscles and joints, and the heart (5).
Joints
A common late stage symptom of Lyme disease is arthritis. This usually occurs after the host has been infected for a few months and leads to triggering the innate and adaptive immune system (6). However in very rare cases, antibiotic-refractory arthritis may occur which is classified as synovitis at the knee that may last for months or years (7). This can be attributed to an individual’s HLDAR alleles that bind a specific OspA epitope leading to a strong Th1 response (6) Patients will typically have a lower count of Foxp3+ regulatory T cells which contributes to the post-treatment duration of arthritis (6). This occurs more frequently in patients with a TLR1 polymorphism (1805GG) that results in an increase in cytokines and chemokines of the affected joints (6). Treatment is usually done with a concoction of various antibiotics such as doxycycline, amoxicillin, and cefuroxime axetil (6).
Cardiovascular System
Lyme carditis is another late stage symptom of lyme disease. It occurs in 4%-10% of patients cand can be fatal if untreated (8). While its mechanism is not well understood, it is hypothesized that B. burgdorferi infects the pericardium/myocardium, resulting in an inflammatory response that leads to Lyme carditis (8). The proposed mechanism for cardiac damage is attributed to the host’s inflammatory response and any cytotoxic effects of the bacteria (8). This may result in the blocking of the atrioventricular (AV) node (9). It is often treated with antibiotics and may even involve a temporary pacemaker may be put inside to avoid high-degree heart block (9).
Central Nervous System
B. burgdorferi is able to penetrate the BBB and infiltrate the mammalian host’s brain. Lyme neuroborreliosis (LNB) targets the central and peripheral nervous system; patients are usually diagnosed with meningitis, cranial neuritis, radiculoneuritis, parenchymal inflammation of the brain and spinal cord, peripheral neuropathy, and encephalopathy (10). The bacteria enters the CNS through the bloodstream or peripheral nervous system and infiltrates the meninges (23). They can be seen in the vascular, perivascular, and extravascular areas of the dura mater; they are also associated with an increase in T cells and leukocytes within the meninges (10). An inflammatory response may be observed coming from glial cells due to an increase in cytokines and chemokines (10). In addition, there is also an increase in IL-6 production by astrocytes and an uptick in oligodendrocyte/neuronal death due to apoptosis and microglial activation respectively (10). Some common symptoms are nerve pain, numbness, double vision, and facial palsy (11).
B. burgdorferi infection may damage neural cells by the direct action of spirochetes or spirochetal products on glial and neuronal cells (1). It is also possible that spirochetes induce cytotoxic or inflammatory mediators locally in glial, neuronal or endothelial cells, and therefore, cause indirect damage (1). Infiltrating immune cells and the presence of cross-reactive antibodies to self-antigens at the site of infection or inflammation may also be harmful to neural cells (1). B. burgdorferi can elicit the production of IL-6, IL-8, and CCL2 in cells of the dorsal root ganglion (DRG) and induce the death of sensory neurons in DRG cell cultures (1).
Cytokine or chemokine signaling and apoptosis are key aspects in the regulation of neuroinflammatory responses (1). Since DRG axons project centrally into the spinal cord and peripherally into the spinal nerves, inflammation and cell death in the DRG elicited by the Lyme disease spirochete could affect neuronal survival and function both in the CNS and PNS (1). Further, as the sensory neurons of the DRG play a key role in the sensation of pain, inflammation in glial and neuronal cells and cell death in the DRG could also influence and modulate the pain response.
Suzanne’s Symptoms
Suzanne is most likely experiencing symptoms induced by Lyme disease in the short term. Her rash, erythema migrans, fever, chills, and fatigue can be attributed to the innate immune response. Specifically, inflammatory cells and their cytokine production results in the symptoms she has experienced so far. Since it has only been a few days, she probably has not experienced arthritis, lyme carditis, and lyme neuroborreliosis as of yet.
References
- Ramesh, G., Santana-Gould, L., Inglis, F. M., England, J. D., & Philipp, M. T. (2013, July 18). The lyme disease spirochete borrelia burgdorferi induces inflammation and apoptosis in cells from dorsal root ganglia - journal of Neuroinflammation. BioMed Central. Retrieved February 9, 2022, from https://jneuroinflammation.biomedcentral.com/articles/10.1186/1742-2094-10-88
- Russell, T. M., Delorey, M. J., & Johnson, B. J. B. (2013, September 16). Borrelia burgdorferi BbHtrA degrades host ECM proteins and stimulates release of inflammatory cytokines in vitro. Wiley Online Library. Retrieved February 9, 2022, from https://onlinelibrary.wiley.com/doi/10.1111/mmi.12377
- CIMB abstract. Lyme Disease Pathogenesis. (n.d.). Retrieved February 9, 2022, from https://www.caister.com/cimb/abstracts/v42/473.html
- Halperin, J. J. (2018). Lyme disease: An evidence-based approach. CABI.
- What Are the Symptoms of Chronic Lyme Disease? [Internet]. IGeneX. 2020 [cited 2022 Feb 11]. Available from: https://igenex.com/tick-talk/what-are-the-symptoms-of-chronic-lyme-disease/
- Arvikar SL, Steere AC. 5. Diagnosis and Treatment of Lyme Arthritis. Infect Dis Clin North Am. 2015 Jun;29(2):269–80.
- Steere AC, Klitz W, Drouin EE, Falk BA, Kwok WW, Nepom GT, et al. Antibiotic-refractory Lyme arthritis is associated with HLA-DR molecules that bind a Borrelia burgdorferi peptide. J Exp Med. 2006 Apr 17;203(4):961–71.
- Grella BA, Patel M, Tadepalli S, Bader CW, Kronhaus K. Lyme Carditis: A Rare Presentation of Sinus Bradycardia Without Any Conduction Defects. Cureus. 11(9):e5554.
- Rivera O, Nookala V. Lyme Carditis. StatPearls [Internet]. 2021 Oct 1 [cited 2022 Feb 12]; Available from: https://www.statpearls.com/ArticleLibrary/viewarticle/50226
- Ford L, Tufts DM. Lyme Neuroborreliosis: Mechanisms of B. burgdorferi Infection of the Nervous System. Brain Sci. 2021 Jun 15;11(6):789.
- How Lyme Disease Affects the Brain and Central Nervous System [Internet]. Verywell Health. [cited 2022 Feb 12]. Available from: https://www.verywellhealth.com/lyme-neuroborreliosis-4581590
4. The Immune Response
i) Host response: what elements of the innate and adaptive (humoral and cellular) immune response are involved in this infection.
In this case, Suzanne had a tick which embedded its head to the back of her calf. Suzanne was then diagnosed with Erythema migrans as she showed one of the first symptoms, resulting from Lyme disease. Lyme disease is caused by the bacterium Borrelia burgdorferi, which is transferred to people through tick bites. Usually, the tick must be attached for roughly 36-48 hours to allow the Lyme disease bacterium to be transmitted [1]. This initial symptoms Suzanne experiences may be due to her body's innate immune response, the first line of defense, that is put into almost immediate action with the presence of B. burgdorferi in the dermis [2]. It is common for invading pathogens to cause inflammation within the body, raising blood flow towards the infected area and causing visible redness, heat, pain, swelling and potential loss of tissue function [3]. Erythema eventually develops as the redness of the rash expands along with the migrating microbe around the site of the tick bite within the host [2]. Erythema migrans is characterised by a circular red region with a clear centre that forms a bulls eye pattern. It can be up to 12 inches broad and warm to the touch, usually not itchy nor painful [4]. Furthermore, fevers, headaches, malaise, myalgia, arthralgia, swollen lymph nodes may also be observed as early symptoms.
Innate immune response:
Innate immunity has different components, such as: physical barriers (tight junctions in the skin, epithelial and mucous membrane surfaces, mucus), anatomical barriers, chemical barriers such as the secretion of antimicrobial peptides (AMPs), soluble mediators; and signaling transduction system such as the complement cascade, and innate cells such as macrophages, dendritic cells, granulocytes, and natural killer cells that attempt to eliminate the pathogen before adaptive immune response come into play [5].
In this bacterial infection, because the tick has bit Suzanne’s leg, the physical barrier of the skin has been breached and the bacteria has directly entered the dermis and into the bloodstream, and the bacteria has started infecting the endothelial cells.
Langerhans cells (LCs)
LCs are the first immune cells encountered by Borrelia burgdorferi. They are found in the epidermis near the dermal interface [6] and express pattern recognition receptors (PRRs) that allow them to recognize pathogens encountered near the skin surface. This leads to the upregulation of MHC class II and migration to lymph nodes to present antigens to lymphocytes [7].
Effector cells
Phagocytic cells, epithelial and endothelial cells, natural killer cells, innate lymphoid cells, and platelets are all examples of effector cells. Granulocytes (neutrophils, eosinophils, basophils, and mast cells), monocytes/macrophages, and dendritic cells make up phagocytic cells [5]. Both phagocytosis and the inflammatory process are carried out by these cells. The majority of cell components have pattern recognition receptors (PRRs) on their cell surfaces and can release cytokines such as IL-6, IL-12, TNF-, and pro-IL1, indicating that they have microbicidal mechanisms. Both the innate and acquired immune systems influence these cells through innate immunity effector pathways [5].
Antigen presenting cells (APCs) include cells such as dendritic cells, monocytes, macrophages, and neutrophils which contain pattern-recognition receptors (PRRs). It is PRRs such as Toll-like receptors (TLRs), and Nucleotide-binding oligomerization domain (NOD)-like receptors such as NOD1 and NOD2 on the host cells that are involved with recognizing B. burgdorferi [8,9].
Macrophages and neutrophils are immune cells that participate in the innate response by responding to many pathogens due to having no specificity. They are quick early responders that rush to the infection site. Both of these cells are powerful killers because they can phagocytose the pathogen and produce extremely damaging chemicals like reactive oxygen species (ROS) or enzymes to destroy it [10]. Neutrophils are recruited by the leukotrienes secreted by macrophages and migrate to the site of infection within 4 hours of infection [11]. They are engaged when their PRRs recognize bacteria and initiate phagocytosis. Most macrophages and neutrophils have PRRs on their surfaces, and when these receptors are activated, these professional phagocytes (including monocytes, eosinophils, and DCs) can phagocytose microbes in their proximity and fuse with lysosomes to form phagolysosomes, which become acidified and kill the bacteria [12]. Despite their similarities to DCs, macrophages have a limited ability to move to lymph nodes. Instead, while phagocytosing a bacterium, their phagolysosomes are able to achieve a significantly lower pH, indicating that they can kill bacteria more effectively [12].
Macrophages are phagocytic and have both an oxygen-dependent and oxygen-independent microbicidal action. They can present antigens and activate lymphocytes, then release and stimulate cytokine production once activated. They also produce extracellular matrix proteins and matrix metalloproteinases (MMP)such as MMP-9, which regulate the immune response and aid tissue remodelling once the inflammatory process has ended [5].
DCs have the ability to transport and carry antigens from peripheral lymphatic nodes to primary lymphatic nodes, where they are presented. These APCs lead to antigen processing and presentation via MHC class II molecules, linking the innate and acquired immune responses [5]. They also play a role in innate immunity against a variety of microbial infections by phagocytosing the pathogen.
Natural killer cells recognise infected cells with reduced MHC molecule expression. The killer cell immunoglobulin-like receptor (KIR) and CD94-NKG2A inhibitory receptors on NK cells identify the MHC class I expressed on the surface of normal host cells, allowing them to discriminate between them [5]. The engagement of these receptors prevents NK cells from lysing and secreting cytokines. NK cells also express PRRs such as TLR-2, -3, -4, -5, -7, and -8 and contain granules with perforins and granzymes that act on target cells to cause lysis or death. NK cells release IFN-y, TNF-a, IL-5, IL-10, IL-13, and chemokines after being activated [5]. These pro-inflammatory cytokines are produced to support T cell differentiation, stimulate macrophage function, promote leukocyte to migrate to the site of infection, and increase MHC expression for better T cell recognition of pathogen [5,30].
Mast cells are located in tissues, mainly in mucosa, and their granules contain heparin, serotonin, and histamine. They may also release a variety of cytokines that enhance the inflammatory process, especially during the early events [5]. They express TLRs and receptors for complement fragments C3a and C5a. When the complement receptors are engaged, mast cells release histamine. Mast cells also have Fc receptors that engage when IgE binds the bacterial antigen, causing the mast cell to degranulate, releasing histamine, protease, and various cytokines. These molecules increase vascular permeability, fluid accumulation & recruit more innate immune cells [7].
Pathogen-associated recognition receptors (PAMP) recognition
The cells of the innate immune system recognize pathogen-associated molecular patterns (PAMPs) on B. burgdorferi’s cell surface through pattern-recognition receptors (PRRs) of the host, including Toll Like Receptors (TLR), Nucleotide Oligomerization Domain (NOD)-like receptors (NLRs), and C-type lectins (CTLs) [13,14]. The recognition between PAMPs and PRRs is necessary before cytokines or chemokines can be produced [14].
Major PAMPs include surface lipoproteins such as outer surface protein A (OspA), OspB and OspC [15]. TLR2 can also form heterodimers with TLR1, allowing it it to recognize a broad range of microbial ligands, such as B. burgdorferi's triacylated lipid element on its cell surface localized lipopeptides [14,16]. TLR1/2 heterodimers are crucial for the recognition and for regulating the outgrowth of Borrelia and resulting inflammation. Borrelia spirochetes lack membrane lipopolysaccharide, suggesting that TLR4 is secondary in the recognition of B. burgdorferi as it requires a certain polysaccharide in order to be activated [14]. However, CD14 is the co-receptor for TLR4 and has been known to exhibit its own pathogen-recognizing capacity [14]. In the context of B. burgdorferi, CD14 on human cells can recognize B. burgdorferi, specifically OspA [17].
TLR2 recognises a PAMP, the peptidoglycan of B. burgdorferi, on host macrophages, which serves as a priming signal for the signaling adaptor MyD88 to be recruited to the plasma membrane for TLR2 activation. MyD88 is crucial for the induction of cytokine production and is activated by Toll-interleukin 1 receptor (TIR) domain-containing adapter protein (TIRAP) [16]. When MyD88 is recruited by TIRAP to the plasma membrane for TLR2 signaling or directly to TLR5, a signaling cascade results [16]. The recruitment of the IRAK kinases, the E3 ubiquitin ligase, TRAF6, and TAK1, as well as the activation of MAP kinases and NF-kB, culminates in a signaling cascade. This signaling pathway then activates pro-inflammatory cytokines such as IL-6, IL-12, TNF-, and pro-IL1. These cytokines can then recruit other components of the innate host immune response and are also involved in the signaling within the adaptive immune system [13].
Other released chemokines found at high levels include CCL2, CCL4, CXCL9, and CXCL10. Importantly, CCL2 and CCL4 are involved with recruiting monocytes and T cells, whereas CXCL9 and CXCL10 are chemoattractant for CD4+ and CD8+ T effector cells to site of infection in Lyme disease [18]. CCL3 and CXCL8 also released and this plays a role in recruiting monocytes, natural killer (NK) cells and T cells, as well as the release of neutrophil respectively [18].
Complement system
One of the most essential enzymatic systems involved in the innate immune response is the complement system. The complement system performs a variety of activities, including inducing an immune response to invading infections, regulating cytokine synthesis, and assisting in the removal of immune complexes and dead or transformed cells [19]. Bacterial pathogens are coated with opsonizing molecules (C1q, C3b, and iC3b) upon entry into the host [20]. The complement system can be activated via 3 pathways; the classical pathway, the lectin pathway and the alternative pathway, all of which can be initiated during a Borrelia infection [14]. C1q, a complement factor, binds to antibodies (such as IgM or IgG) on the bacterial surface, activating the complement cascade and ultimately the complement components C2 and C4 via the classical pathway. The lectin pathway is initiated by lectin binding to carbohydrates on B. burgdorferi’s surface, and the alternative pathway is initiated by the spontaneous activation of C3 on the bacterial surface. These pathways result in the formation of C3 and C5 convertases and the formation of the membrane attack complex (MAC), ultimately leading to cell lysis. This is due to the C3 covalently binding to proteins expressed by B. burgdorferi and causing the bacterium to be opsonized. The opsonized Borrelia is then recognized by complement receptor (CR)1, CR2 and CR3 on phagocytic cells, thus leading to its destruction [14].
Mannose-binding lectin (MBL) protein
MBL proteins are largely produced in the liver and then released into the bloodstream. They can identify carbohydrates that function as opsonins and bind and activate complement factors such C1q, increasing the inflammatory response [5]. MASP is made up of two genes and five gene products. MASP-1, MASP-3, and MAP-1 are MASP-1/3 gene alternative splice products, whereas MASP-2 and sMAP are MASP-2 gene alternative splice products [5]. MASPs form complexes with MBL, and MBL binding to carbohydrate ligands is hypothesised to cause conformational changes in the associated MASP, which boost proteolytic activity [5]. The alternative route and the lectin complement pathway have both been found to be activated by MASP-1 and MASP-2 [5].
Reactive oxygen species (ROS)
Mammalian cells, particularly phagocytes, produce reactive oxygen species (ROS) and reactive oxygen intermediates (ROI) in response to a variety of microbial pathogens [5]. These molecules are generated by activation of the enzymatic complex nicotinamide adenine dinucleotide phosphate (NADPH) oxidase (NOX2) and include superoxide anion (O2-), hydrogen peroxide (H2O2), hydroxyl radical (·OH), peroxynitrite (ONOO-),hypochlorous acid (-OCl), etc. Both ROS and ROI are known to play diverse roles in inflammation, host defense, and homeostasis [5].
One of the most prominent RNIs is nitric oxide (NO), which is created via an oxidative process involving the catabolism of L-arginine [5]. NO generation by inducible nitric oxide synthase (iNOS) is one of the most important microbicidal strategies used by phagocytic cells against a variety of pathogens [5]. iNOS is expressed by immune cells such as macrophages, neutrophils, dendritic cells, and NK cells, and may be triggered by a variety of stimuli such as IFN-y, TNF-a, and LPS. NO, like ROS, may play a role in inflammation and its control [5].
Antimicrobial peptides (AMPs)
Innate and epithelial cells, including keratinocytes, release AMPs, which are host defence peptides. Their antibacterial action is wide, with fungus, bacteria, and viruses being the most common targets. During an infection or damage, they can be found constitutively or can be produced following activation of the host cells through multiple PRRs. These AMPs are also engaged in a variety of cell activities, including cell migration, proliferation, differentiation, cytokine production, angiogenesis, and wound healing, among others [5].
Neutrophils and epithelial cells both produce cathelicidin, also known as LL-37. Gram-negative and Gram-positive bacteria, fungi, and viruses are all susceptible to this AMP. It generates an immunological response, which leads to the recruitment of inflammatory cells and the production of cytokines by host cells [5].
Defensins include α- and β-defensins. α-defensins (hαD-1, -2, -3, -4) are stored in the azurophil granules of neutrophils, and HαD-5 and -6 are synthesised by the Paneth cells in the gastrointestinal tract [3]. Keratinocytes generate the majority of B-defensins (hD-1, -2, -3). Defensins, like cethelicidins, have antibacterial properties and are chemotactic, inducing the production of cytokines and chemokines [5].
Extracellular traps
Extracellular DNA traps are involved with viral processes, allergy and autoimmune illnesses, and are part of innate immunity. Different leukocytes, such as neutrophils, eosinophils, monocytes, and mast cells, produce these structures. They are referred to as NETs, EETs, METs, and MCETs [5]. DNA, histones, and the contents of intracellular granules such as elastase, myeloperoxidase (MPO), cathelicidins, tryptase, cationic proteins, and major basic protein, among others, make up extracellular traps [5]. The granulocyte/macrophage-colony stimulating factor (GM-CSF), interferons, IL-8, C5a, and LPS all trigger these traps. Extracellular traps can bind to and destroy microbial infections once they've been produced. These DNA traps might play a role in the progression of autoimmune and chronic inflammatory disorders [5].
Inflammation
Inflammation is a general reaction by the host to an infectious, physical, or chemical harm that involves the migration of leukocytes and plasma proteins from the peripheral circulation to the site of injury or tissue damage. Redness, swelling, heat, pain, and loss of tissue function are all indications of inflammation. Blood flow and vascular permeability both rise during this process, primarily in the vascular endothelium at the local level. Endothelial cell retraction causes vascular permeability, which allows leukocytes to pass through and plasmatic proteins including complement, coagulation factors, and antibodies such as IgG to pass through. Inflammation is the cause of Suzanne's rash feeling "hot to touch" and "burning."
Suzanne’s innate immune response:
At site of tissue damage, mast cells release histamine and inflammatory molecules to cause local blood vessel dilation, resulting in increase of blood flow to the area. This results in Increased blood flow, further causing inflammation. In early innate responses, complement proteins from blood enter tissues and kill bacteria. Resident macrophages recognize PAMPs with PRRs and phagocytose bacteria, make TNF-α, IL-1 and IL-6 to start induced innate responses. In induced innate responses, cytokines released by macrophages act on blood vessel walls, increasing permeability and inducing expression of adhesion molecules on surface of endothelial cells lining blood vessel. Cytokines also act on bone marrow to increase monocyte and neutrophil production. The increased permeability of blood vessels allows components of blood (Ex. fluid, complement proteins, coagulation proteins, antibodies, neutrophils, monocytes) to leak into tissue, and the accumulated fluid cause swelling and pain. Adhesion molecules allow neutrophils and monocytes to adhere to endothelial cells of blood vessel and extravasate through tight junctions of endothelial cells. The chemogradient guides neutrophils, monocytes and DCs to location of bacteria. If this is not enough to kill bacteria, adaptive response is required.
Adaptive response:
Whereas the innate immune response is usually the first line of defense at play within the host, the adaptive immune response is more complex, acting as the host's second line of defense. If B. burgdorferi is able to overcome the components of the innate immune system, then the adaptive immune response is engaged. The adaptive immune responses contain two main adaptive responses, consisting of the humoral immune response as well as the cellular immune response. Dendritic cells and macrophages both have the ability to initiate an adaptive immune response through digesting and presenting the antigen on its surface with its Major Histocompatibility Complex (MHC) class I or II [7]. When a dendritic cell picks up a virus from infected tissue, it activates and goes to a lymph node nearby. Dendritic cells migrate towards the T cell zones and present antigens to initiate T cell responses, whereas macrophages mainly stay in tissues at the site of infection to initiate localized inflammatory response and phagocytose microbes during infection by B. burgdorferi [7]. The dendritic cell grows into a highly efficient antigen-presenting cell (APC) after activation and undergoes modifications that allow it to activate pathogen-specific lymphocytes in the lymph node [21]. The classical and lectin pathways are also active to develop specific antibodies in response to the pathogen and activate MBL-associated proteases (MASPs) respectively [22].
MHC I and II proteins play a pivotal role in the adaptive branch of the immune system because both classes of proteins share the task of presenting peptides on the cell surface for recognition by T cells [23]. CD4+ T helper cells become activated once they bind to MHC II on dendritic cell surfaces via T cell receptors (TCR), and CD8+ cytotoxic T lymphocytes cells become activated once they bind to MHC I on DCs via TCRs [23]. The activation of T cells is needed for the activation of the adaptive immune system.
Humoral Adaptive Response:
Humoral immunity relies on the freely circulating antibodies produced by B cells interacting with antigens on B.burgdorferi to bind, neutralize and mark for phagocytosis [24]. B cells produce antibodies against the specific antigen to bind to them and neutralize or cause phagocytosis of them. Specifically, it has been found that immunoglobulin M (IgM) and IgG antibodies respond to B. burgdorferi antigens such as OspA, OspC and flagellar antigen B (FlaB) [25]. This mechanism functions to protect the extracellular spaces of the host by producing antigens from activated B cells in the lymph nodes [26]. Once there, B. burgdorferi causes enlargement of the lymph nodes due to rapid B cell proliferation, some of which can then produce antibodies specific for B. burgdorferi [26]. High levels of B. burgdorferi antigen-specific antibodies are produced during infection and they have the ability to prevent reinfection with the same B. burgdorferi strains. The antibody response also results in reduction, but not elimination, of B. burgdorferi from tissues. Both T-independent and T-dependent antigens are targeted by the humoral immune response, representing a wide variety of surface proteins with different functions [27]. However, because B. burgdorferi can successfully evade and disarm the host’s humoral and cell-mediated immune mechanisms, antibodies and memory cells do not typically remain in the host for more than a few weeks post infection [26].
Differentiation of T cells into its effector cell type, such as CD4 T follicular helper cells (Tfh), CD4 T helper 1 cells (Th1), or CD8 cytotoxic T cells. Tfh cells, along with follicular dendritic cells (FDCs) are essential for the formation of germinal centers, which is where B cells undergo proliferation, class-switching, affinity maturation, and production of long-lived memory cells [28]. These cells can undergo class-switching to express IgG, however, IgG-plasma cells accumulate very slowly in the bone marrow and only 50% are due to T-dependent responses [29], which is atypical for bacterial infections. IgG does not appear to contribute significantly to the long-term Ab responses. This may be due to the formation of only transient germinal centers that are unable to sustain B cell maturation and development into memory cells [30]. This contributes to the persistence of B. burgdorferi in the host as well as host susceptibility to reinfection.
Cellular Adaptive Response:
The cellular adaptive immune response involves CD4+ which are important for B cell activation and antibody production specific against B. burgdorferi. By releasing effector chemicals that can activate phagocytes, CD4+ T cells help to protect against B. burgdorferi infections. IFN and tumour necrosis factor-alpha (TNF) are the major effector molecules thought to be involved in CD4+ T cell-mediated defence against intracellular infections [31]. Infection with B. burgdorferi causes CD4+ T cells to develop into T Helper 1 (TH1) cells, which generate IFN and TNF, and the induction of TH1 cells is mediated by IL-12 production by DCs. When infected with B. burgdorferi, CD4+ T cells differentiate into T Helper 17 (TH17) cells in the absence of external IFN [31]. Inflammatory cytokines such as IL-17A, IL-22, and TNF are released by these cells. The combination of IL-17A and TNF can then cause macrophages to produce and release NO and ROS. The synthesis of chemokines is induced by IL-17A, which leads to the recruitment of neutrophils, which contribute to local inflammation. IL-22 does not act on immune cells, but rather on numerous tissue cells to cause the production of antimicrobial peptides, which helps to eliminate germs [32]. Many of the pro-inflammatory cytokines produced are responsible for Suzanne's symptoms, including her fever.
References:
1. CDC. Transmission [Internet]. Centers for Disease Control and Prevention. 2020 [cited 2022 Feb 18]. Available from: https://www.cdc.gov/lyme/transmission/index.html
2. The bulls-eye rash of Lyme disease: Investigating the cutaneous host-pathogen dynamics of erythema migrans [Internet]. ASM.org. 2018 [cited 2022 Feb 18]. Available from: https://asm.org/Articles/2018/April/going-skin-deep-investigating-the-cutaneous-host-p
3. Chen L, Deng H, Cui H, Fang J, Zuo Z, Deng J, et al. Inflammatory responses and inflammation-associated diseases in organs. Oncotarget [Internet]. 2018 [cited 2022 Feb 18];9(6):7204–18. Available from: https://www.ncbi.nlm.nih.gov/labs/pmc/articles/PMC5805548/
4. What to know about erythema migrans [Internet]. WebMD. [cited 2022 Feb 18]. Available from: https://www.webmd.com/skin-problems-and-treatments/what-to-know-erythema-migrans
5. Aristizábal B, González Á. Innate immune system. El Rosario University Press; 2013.
6. Kaplan DH. Ontogeny and function of murine epidermal Langerhans cells. Nat Immunol [Internet]. 2017 [cited 2022 Feb 18];18(10):1068–75. Available from: https://pubmed.ncbi.nlm.nih.gov/28926543/
7. Bockenstedt LK, Wooten RM, Baumgarth N. Immune response to Borrelia: Lessons from Lyme disease spirochetes. Curr Issues Mol Biol [Internet]. 2021 [cited 2022 Feb 18];42:145–90. Available from: https://pubmed.ncbi.nlm.nih.gov/33289684/
8. Skogman BH, Hellberg S, Ekerfelt C, Jenmalm MC, Forsberg P, Ludvigsson J, et al. Adaptive and innate immune responsiveness to Borrelia burgdorferi sensu lato in exposed asymptomatic children and children with previous clinical Lyme borreliosis. Clin Dev Immunol [Internet]. 2012 [cited 2022 Feb 18];2012:294587. Available from: https://www.hindawi.com/journals/jir/2012/294587/
9. Tkáčová Z, Bhide K, Mochnáčová E, Petroušková P, Hruškovicová J, Kulkarni A, et al. Comprehensive mapping of the cell response to Borrelia bavariensis in the brain microvascular endothelial cells in vitro using RNA-seq. Front Microbiol [Internet]. 2021;12:760627. Available from: http://dx.doi.org/10.3389/fmicb.2021.760627
10. Nguyen GT, Green ER, Mecsas J. Neutrophils to the ROScue: Mechanisms of NADPH oxidase activation and bacterial resistance. Front Cell Infect Microbiol [Internet]. 2017;7. Available from: http://dx.doi.org/10.3389/fcimb.2017.00373
11. Xu Q, Seemanapalli SV, Reif KE, Brown CR, Liang FT. Increasing the recruitment of neutrophils to the site of infection dramatically attenuates Borrelia burgdorferi infectivity. J Immunol [Internet]. 2007 [cited 2022 Feb 18];178(8):5109–15. Available from: https://pubmed.ncbi.nlm.nih.gov/17404293/
12. Uribe-Querol E, Rosales C. Phagocytosis: Our current understanding of a universal biological process. Front Immunol [Internet]. 2020;11:1066. Available from: http://dx.doi.org/10.3389/fimmu.2020.01066
13. Medzhitov R, Janeway C Jr. The Toll receptor family and microbial recognition. Trends Microbiol [Internet]. 2000 [cited 2022 Feb 18];8(10):452–6. Available from: https://pubmed.ncbi.nlm.nih.gov/11044679/
14. Oosting M, Buffen K, van der Meer JWM, Netea MG, Joosten LAB. Innate immunity networks during infection with Borrelia burgdorferi. Crit Rev Microbiol [Internet]. 2016 [cited 2022 Feb 18];42(2):233–44. Available from: https://pubmed.ncbi.nlm.nih.gov/24963691/
15. Cervantes JL, Hawley KL, Benjamin SJ, Weinerman B, Luu SM, Salazar JC. Phagosomal TLR signaling upon Borrelia burgdorferi infection. Front Cell Infect Microbiol [Internet]. 2014 [cited 2022 Feb 18];4:55. Available from: https://www.ncbi.nlm.nih.gov/labs/pmc/articles/PMC4033037/
16. Petnicki-Ocwieja T, Kern A. Mechanisms of Borrelia burgdorferi internalization and intracellular innate immune signaling. Front Cell Infect Microbiol [Internet]. 2014;4:175. Available from: http://dx.doi.org/10.3389/fcimb.2014.00175
17. Giambartolomei GH, Dennis VA, Lasater BL, Philipp MT. Induction of pro- and anti-inflammatory cytokines by Borrelia burgdorferi lipoproteins in monocytes is mediated by CD14. Infect Immun [Internet]. 1999 [cited 2022 Feb 18];67(1):140–7. Available from: https://pubmed.ncbi.nlm.nih.gov/9864208/
18. Shin JJ, Strle K, Glickstein LJ, Luster AD, Steere AC. Borrelia burgdorferi stimulation of chemokine secretion by cells of monocyte lineage in patients with Lyme arthritis. Arthritis Res Ther [Internet]. 2010;12(5):R168. Available from: http://dx.doi.org/10.1186/ar3128
19. Parra-Medina R, Quintero-Ronderos P, Rodríguez ÉG. The complement system. El Rosario University Press; 2013.
20. Singh SK, Girschick HJ. Molecular survival strategies of the Lyme disease spirochete Borrelia burgdorferi. Lancet Infect Dis [Internet]. 2004 [cited 2022 Feb 18];4(9):575–83. Available from: https://pubmed.ncbi.nlm.nih.gov/15336225/
21. Janeway CA Jr, Travers P, Walport M, Shlomchik MJ. Principles of innate and adaptive immunity. London, England: Garland Science; 2001.
22. Coburn J, Garcia B, Hu LT, Jewett MW, Kraiczy P, Norris SJ, et al. Lyme disease pathogenesis. Curr Issues Mol Biol [Internet]. 2021 [cited 2022 Feb 18];42:473–518. Available from: https://www.ncbi.nlm.nih.gov/labs/pmc/articles/PMC8046170/
23. Gair CMA, Jane MCA. 23.2. Adaptive immune response. In: Concepts of Biology-1st Canadian Edition Molnar Class. 2015.
24. Adaptive immunity – humoral and cellular immunity [Internet]. Healio.com. [cited 2022 Feb 18]. Available from: https://www.healio.com/hematology-oncology/learn-immuno-oncology/the-immune-system/adaptive-immunity-humoral-and-cellular-immunity
25. Vaz A, Glickstein L, Field JA, McHugh G, Sikand VK, Damle N, et al. Cellular and humoral immune responses to Borrelia burgdorferi antigens in patients with culture-positive early Lyme disease. Infect Immun [Internet]. 2001 [cited 2022 Feb 18];69(12):7437–44. Available from: https://www.ncbi.nlm.nih.gov/labs/pmc/articles/PMC98832/
26. Anderson C, Brissette CA. The brilliance of Borrelia: Mechanisms of host immune evasion by Lyme disease-causing spirochetes. Pathogens [Internet]. 2021 [cited 2022 Feb 18];10(3):281. Available from: https://www.ncbi.nlm.nih.gov/labs/pmc/articles/PMC8001052/
27. Tracy KE, Baumgarth N. Borrelia burgdorferi manipulates innate and adaptive immunity to establish persistence in rodent reservoir hosts. Front Immunol [Internet]. 2017 [cited 2022 Feb 18];8:116. Available from: https://pubmed.ncbi.nlm.nih.gov/28265270/
28. Ramsey ME, Hyde JA, Medina-Perez DN, Lin T, Gao L, Lundt ME, et al. A high-throughput genetic screen identifies previously uncharacterized Borrelia burgdorferi genes important for resistance against reactive oxygen and nitrogen species. PLoS Pathog [Internet]. 2017;13(2):e1006225. Available from: http://dx.doi.org/10.1371/journal.ppat.1006225
29. Hastey CJ, Elsner RA, Barthold SW, Baumgarth N. Delays and diversions mark the development of B cell responses to Borrelia burgdorferi infection. J Immunol [Internet]. 2012 [cited 2022 Feb 18];188(11):5612–22. Available from: https://pubmed.ncbi.nlm.nih.gov/22547698/
30. Elsner RA, Hastey CJ, Baumgarth N. CD4+ T cells promote antibody production but not sustained affinity maturation during Borrelia burgdorferi infection. Infect Immun [Internet]. 2015 [cited 2022 Feb 18];83(1):48–56. Available from: https://www.ncbi.nlm.nih.gov/labs/pmc/articles/PMC4288900/
31. Nicholson LB. The immune system. Essays Biochem [Internet]. 2016 [cited 2022 Feb 18];60(3):275–301. Available from: https://portlandpress.com/essaysbiochem/article/60/3/275/78223/The-immune-system
32. Dixon BREA, Radin JN, Piazuelo MB, Contreras DC, Algood HMS. IL-17a and IL-22 induce expression of antimicrobials in gastrointestinal epithelial cells and may contribute to epithelial cell defense against Helicobacter pylori. PLoS One [Internet]. 2016 [cited 2022 Feb 18];11(2):e0148514. Available from: https://www.ncbi.nlm.nih.gov/labs/pmc/articles/PMC4750979/
ii) Host damage: what damage ensues to the host from the immune response?
Effects on the Cardiovascular System
Lyme carditis can occur when B. burgdorferi is spread to the blood and infects the heart tissues (1). It is primarily caused by the inflammation of the host's immune response to the bacterial infection that causes tissue damage. B. burgdorferi can disrupt the normal electrical signaling of the heart at the atrioventricular level, leading to defects in the coordination of heart contractions (2, 3). Mild heart block can then occurs, in which there is an impairment of electrical signal that controls contraction of the upper and lower chambers of the heart (1). Ultimately, this can result in symptoms such as shortness of breath, fainting, chest pains, light-headedness and palpitations (1). This generally is self-limited and permanent heart block is rare. The patient may need temporary pacing to alleviate the effects (4).
The production of reactive oxygen species (ROS) by neutrophils and macrophages causes oxidative stress. This is caused by the immune response's mix of IFN-y and TNF-a [5]. The ROS generated by endothelium cells infected with B. burgdorferi can, however, contribute to harm via oxidative stress due to the buildup of ROS. [5]. As a result, ROS and RNS produced by the combination of TNF and IFN serve as both a host defensive mechanism and a mechanism for infection-induced damage. In the course of infection spread, these molecules induce changes in blood consistency as well as harm to adjacent host cells [6].While ROS and RNS can aid in the killing of B. burgdorferi, they can also induce oxidative damage to host cell DNA, proteins, and lipids. There is increased vascular permeability of blood vessels and increased blood flow to the site of inflammation during inflammation, which ultimately leads to damage and destruction of host tissues [5].
Effects on the Nervous System
Lyme disease may also affect the central nervous system, in which the inflammation in response to infection may result in slower thinking, difficulty with concentration, difficulty with remembering, and in rare cases, Bell's palsy in untreated individuals (7). Fortunately, this can be treated effectively with the use of antibiotics (7).
A “classic triad” exists to describe the three nervous tissue pathologies that occur with untreated or prolonged B. burgdorferi infection. These include lymphocytic meningitis, radiculoneuritis, and cranial neuritis (8). The meningeal lining or brain parenchyma can be damaged due to the inflammation of peripheral nerves due to B. burgdorferi. Plasma cells and lymphocytes can then infiltrate these tissues, specifically in the dorsal root ganglia, nerve roots, and gray matter of the central nervous system components, the brain and the spinal cord (9). This occurs when the spirochete binds to the blood vessel endothelium and triggers an inflammation cascade in perivascular tissue. The damage to the blood–brain barrier can also then be damaged if B. burgdorferi penetrates into the CNS (9). B. burgdorferi can affect both the peripheral and central nervous systems; this is called Lyme neuroborreliosis. If the cranial nerves are affected by bacterial invasion, facial palsy may also be observed. If the peripheral nerves are affected, patients may experience radiculoneuritis in which patients feel numbness, tingling, pain, or weakness in their arms and legs (10). If the central nervous system is affected, the patient may experience fever, headaches, light sensitivity, and a stiff neck (11). Histologically, nerve lesions are observed and show axonal injury where there is an accumulation of lymphocytes and plasma cells around epineural blood vessels due to immunological responses triggered by the bacteria (12).
Persisting levels of high IL-6 cause persistent high concentration of SAA which in term can lead to chronic inflammatory diseases. This process in the long run causes additional damage and weakening of various organs. Various roles of IL-6 in relation to iron transfer could further cause hypoferremia and anemia in individuals with chronic inflammation (13). Likewise, after initial infection in the second stage of Lyme disease, B. burgdorferi spreads to secondary organs (14).
Chronic inflammation
Chronic inflammation is one risk. Lyme arthritis develops when B. burgdorferi penetrates joint tissue and causes inflammation, and individuals who do not receive immediate treatment may experience persistent inflammation in the region. Chronic inflammation is sometimes referred to as slow, long-term inflammation that lasts for several months to years [15]. There is increased vascular permeability of blood vessels and increased blood flow to the site of inflammation during inflammation. Inflammation also requires a large amount of metabolic energy and frequently leads in damage and destruction of host tissues [5].
Oxidative stress
The production of reactive oxygen species (ROS) by neutrophils and macrophages causes oxidative stress. This is caused by the immune response's mix of IFN-y and TNF-a [5]. The ROS generated by endothelium cells infected with B. burgdorferi can, however, contribute to harm via oxidative stress due to the buildup of ROS [5]. As a result, ROS and RNS produced by the combination of TNF and IFN serve as both a host defensive mechanism and a mechanism for infection-induced damage. In the course of infection spread, these molecules induce changes in blood consistency as well as harm to adjacent host cells [6]. While ROS and RNS can aid in the killing of B. burgdorferi, they can also induce oxidative damage to host cell DNA, proteins, and lipids, which can lead to inflammation and cell death [6]. Overall, the immunological response to infection causes oxidative stress-mediated endothelium injury, which can lead to endothelial cell death, which compromises host endothelial integrity [6].
Rash & skin/organ damage
Dilation of blood vessels and increased blood flow into the surrounding tissues of damage cause a rash [3], as seen in Suzanne’s case (3). As the pathogen disseminates throughout the bloodstream, it will induce inflammatory responses throughout the host organs and skin which contribute to a spotted skin rash.
Erythema migrans occurs in approximately 70-80% of patients infected with B. burgdorferi. It begins at the site of the tick bite after 3-30 days (16). When the tick carrying B. burgdorferi bites the skin, the host innate immune response is initiated and two inflammatory reactions ensue (17), leading to the bullseye rash observation. As the tick bites down, the immune response towards the salivary proteins of the tick causes the central rash, which is stationary. The bacteria carried by the tick causes a separate immune response. As the bacteria replicate locally and spread outwards from the point of entry (tick bit site), the rash expands, developing the outer circle observed in erythema migrans (17).
Host immune responses to B. burgdorferi lipoproteins induces the release of host enzymes, resulting in the digestion of extracellular matrix proteins, allowing bacteria to move from the site of entry towards other tissues. As it expands, the bacteria stimulate the release of inflammatory cytokines as well as macrophages to phagocytose the pathogen, which leads to the migrating rash observed (18). The bullseye pattern is produced due to rapid macrophage clearance from the infection site. Slower macrophage clearance from the tissues would lead to a more homogenous rash (17) instead of the central clearing rash observed in erythema migrans.
If left untreated, infection can spread to joints, the heart, and the nervous system [19].When the bacteria reach these areas, they again will induce an inflammatory and oxidative-stress response within the organs that can lead to its damage within the host. The final and most severe stage of Lyme disease is characterized by arthritis at the lower joints, which can be accompanied by carditis and neuropathy (20). Lyme arthritis develops around 1-2 post-infection, and the patient may experience joint swelling and/or pain, most commonly the knees, as well as the shoulder, ankle, elbow, jaw, wrist, and hip (21). The bacterial dissemination from the point of entry to the synovial tissue initiates the inflammation of the joint. The bacteria invasion leads to the recruitment of mononuclear cells into the infected joint tissue, leading to neutrophil, complement, and cytokine accumulation in the synovial fluid (22). This is due to the recognition of bacterial PAMPs by host TLR2 and MyD88 (23). The bacteria also recruits host matrix metalloproteinases, which may be the cause of joint erosion in long-lasting infections (24).
More rarely, severe neuropathy is reported. The patient may develop chronic encephalomyelitis where they experience spastic paraparesis and/or cognitive impairment (25). The patient may also develop stroke-like symptoms (26). However, there are still some debates on whether these symptoms can be fully attributed to Lyme disease.
References:
1. Krause PJ, Bockenstedt LK. Cardiology patient pages. Lyme disease and the heart. Circulation [Internet]. 2013;127(7):e451-4. Available from: http://dx.doi.org/10.1161/CIRCULATIONAHA.112.101485
2. CDC Editors. Signs and symptoms of Lyme disease | CDC [Internet]. Centers for Disease Control and Prevention. 2021 [cited 11 February 2022]. Available from: https://www.cdc.gov/lyme/signs_symptoms/index.html
3. Steere A. Lyme Carditis: Cardiac Abnormalities of Lyme Disease. Annals of Internal Medicine. 1980;93:8-16.
4. Pinto D. Cardiac manifestations of lyme disease. Medical Clinics of North America. 2002;86(2):285-296.
5. Aristizábal B, González Á. Innate immune system. El Rosario University Press; 2013.
6. Pizzino G, Irrera N, Cucinotta M, Pallio G, Mannino F, Arcoraci V, et al. Oxidative stress: Harms and benefits for human health. Oxid Med Cell Longev [Internet]. 2017 [cited 2022 Feb 13];2017:1–13. Available from: https://www.ncbi.nlm.nih.gov/labs/pmc/articles/PMC5551541/
7. ALDF. Does Lyme disease affect the brain and nervous system? [Internet]. Aldf.com. [cited 2022 Feb 13]. Available from: https://www.aldf.com/does-lyme-disease-affect-the-brain-and-nervous-system-2/
8. Halperin JJ. Chronic Lyme disease: misconceptions and challenges for patient management. Infection and Drug Resistance. 2015;8:119.
9. Fallon BA, Levin ES, Schweitzer PJ, Hardesty D. Inflammation and central nervous system Lyme disease. Neurobiology of disease. 2010 Mar 1;37(3):534-41.
10. Pachner A, Steere A. The triad of neurologic manifestations of Lyme disease: Meningitis, cranial neuritis, and radiculoneuritis. Neurology. 1985;35(1):47-47.
11. CDC Editors. Neurologic Lyme disease | CDC [Internet]. Centers for Disease Control and Prevention. 2022 [cited 11 February 2022]. Available from: https://www.cdc.gov/lyme/treatment/NeurologicLyme.html
12. Vallat J, Hugon J, Lubeau M, Leboutet M, Dumas M, Desproges-Gotteron R. Tick-bite meningoradiculoneuritis: Clinical, electrophysiologic, and histologic findings in 10 cases. Neurology. 1987;37(5):749-749.
13. Tanaka T, Narazaki M, Kishimoto T. IL-6 in inflammation, immunity, and disease. Cold Spring Harbor perspectives in biology. 2014 Oct 1;6(10):a016295.
14. Rupprecht TA, Koedel U, Fingerle V, Pfister HW. The pathogenesis of lyme neuroborreliosis: from infection to inflammation. Molecular medicine. 2008 Mar;14(3):205-12.
15. Pahwa R, Goyal A, Bansal P, Jialal I. Chronic Inflammation. StatPearls Publishing; 2021.
16. CDC. Signs and symptoms of Lyme disease [Internet]. Centers for Disease Control and Prevention. 2021. Available from: https://www.cdc.gov/lyme/signs_symptoms/index.html
17. Hagen A. The Bulls-Eye Rash of Lyme Disease: Investigating the Cutaneous Host-Pathogen Dynamics of Erythema Migrans [Internet]. American Society for Microbiology. 2018 [cited 18 February 2022]. Available from: https://asm.org/Articles/2018/April/going-skin-deep-investigating-the-cutaneous-host
18. Hu L, Eskildsen M, Masgala C, Steere A, Arner E, Pratta M et al. Host metalloproteinases in Lyme arthritis. Arthritis & Rheumatism. 2001;44(6):1401-1410.
19. Uribe-Querol E, Rosales C. Phagocytosis: our current understanding of a universal biological process. Frontiers in immunology. 2020 Jun 2;11:1066.
20. Petnicki-Ocwieja T, Kern A. Mechanisms of Borrelia burgdorferi internalization and intracellular innate immune signaling. Frontiers in Cellular and Infection Microbiology. 2014;4:175.
21. Lyme arthritis | CDC [Internet]. Centers for Disease Control and Prevention. 2021 [cited 18 February 2022]. Available from: https://www.cdc.gov/lyme/treatment/LymeArthritis.html
22. Puius Y, Kalish R. Lyme Arthritis: Pathogenesis, Clinical Presentation, and Management. Infectious Disease Clinics of North America. 2008;22(2):289-300.
23. Guerau-de-Arellano M, Huber B. Chemokines and Toll-like receptors in Lyme disease pathogenesis. Trends in Molecular Medicine. 2005;11(3):114-120.
24. Behera A, Hildebrand E, Scagliotti J, Steere A, Hu L. Induction of Host Matrix Metalloproteinases by Borrelia burgdorferi Differs in Human and Murine Lyme Arthritis. Infection and Immunity. 2005;73(1):126-134.
25. Oschmann P, Dorndorf W, Hornig C, Schäfer C, Wellensiek H, Pflughaupt K. Stages and syndromes of neuroborreliosis. Journal of Neurology. 1998;245(5):262-272.
26. Reik L. Stroke due to Lyme disease. Neurology. 1993;43(12):2705-2705.
iii) Bacterial evasion: how does the bacteria attempt to evade these host response elements.
B. burgdorferi have developed multiple mechanisms to evade the host's immune system and response elements. It has been discovered that the bacteria can cause localized immunosuppression through exploitation of the IFN pathways so that the function of T-lymphocytes is inhibited (1). In addition to immunosuppression, it can also break down connective tissues through lowering connective tissues adhesion so that bacteria can easily swim and invade the skin (1). In this manner, B. burgdorferi essentially enables itself to better survive, proliferate and infect the host whilst suppressing and evading the host's innate and adaptive immune responses (2). The bacterial virulence factor, BBK32 of B. burgdorferi, is an adhesin that allows it to slow its movement, enter the vasculature, and get into the blood of the host more easily so that it can target and colonize within joint tissues (3).
Tick saliva contains proteins that are able to inhibit the complement system (4). Salp15, a tick salivary protein, binds to outer surface protein OspC to protect the bacterium from antibody-mediated killing (5). This occurs because Salp15 inhibits a portion of the membrane attack complex (MAC) to be added, thereby preventing the MAC from assembling correctly (6). Salp20, another tick salivary protein, can inhibit the complement alternative pathway through the dissociation of the C3 convertase components by binding and dissociating C3BbP, the active form of C3 convertase (4). Similarly, the Tick Salivary Lectin Pathway Inhibitor (TSLPI) is a dominant complement inhibitor in tick saliva. TSLPI reduces complement-mediated killing by interfering with the complement lectin pathway cascade through obstruction of the mannose-binding lectin (MBL)-dependent C4 activation. This interference results in impaired neutrophil phagocytosis and chemotaxis and diminished lysis of B. burgdorferi (4).
B. burgdorferi has demonstrated resistance to antimicrobial proteins and a limited susceptibility to lysosomes. The resistance to lactoferrin, an iron-binding and transport protein is partly due to the fact that B. burgdorferi does not require iron for survival (7).
B. burgdorferi can interfere with phagocyte activities by causing an increase in the production of anti-inflammatory interleukin IL-10 (6). IL-10 suppresses the secretion of proinflammatory cytokines TNFα, IL-6, and IL-12 produced by macrophages and dendritic cells. IL-10 production also leads to suppression of phagocytosis by macrophages and a decrease in the production of proinflammatory mediators and co-stimulatory molecules in antigen-presenting cells (8).
Phagocytes such as macrophages and dendritic cells kill bacteria through the production of reactive oxygen species (ROS) or reactive nitrogen species (RNS), which is able to damage bacteria DNA and proteins. B. burgdoferi encodes genes that provide resistance to ROS and RNS for important proteins such as DNA repair enzymes, ribonuclease, and transport proteins (9,10). 66 genes have been identified to confer resistance to ROS and RNS, for example, uvrB and uvrC is needed for nucleotide excision repair in exposure to RNS (10). Furthermore, unlike many other bacteria, B. burgdorferi used a manganese cofactor rather than iron so that DNA damage is less likely to occur and B. burgdorferi essentially has immunity against the host sequestering nutrients such as iron (2).
The complement system enhances the phagocytosis, opsonization, and lysis of the invading bacteria. Complement can be activated through the classical, lectin, or alternative pathways. Complement is abundant in the blood, which B. burgdorferi needs to overcome when traveling in blood to infect other tissues. B. burgdorferi outer surface proteins can inhibit the complement cascade at its initiation, amplification, or terminal steps. They function by either recruiting endogenous complement regulators, recruiting endogenous proteases and inhibiting their proteolysis activity of complement proteins, or binding complement proteins directly to inhibit its activity (11,12).
- BBK32 binds to C1r and traps C1 in a zymogen state (11). C1r is one of the components of the C1 complex, which functions at the initiation of the classical pathway where it cleaves downstream C4 and C2 and the cascade ensues. BBK32 prevents C1 complex formation, thus inhibiting the early stages of the classical pathway.
- OspC binds C4b and interferes with the activation of both the classical pathway and lectin pathway. This binding to C4b prevents the formation of the C3 proconvertase as it is composed of C4b and C2b fragments (12).
- Bacterial P43 recruits C4b-binding protein (C4BP), downregulating the classical pathway and lectin pathway (11) as there is a decrease in the availability of C4b to form C3 convertase with C2b fragments.
- CspA and CspZ bind factor H (FH) and factor H-like protein 1 (FHL-1), which are negative regulators that inhibit activation of the alternative complement pathway. This binding of FH/FHL-1 on the surface of B. burgdorferi prevents complement deposition on the surface of the bacteria, thus evading complement-mediated opsonization (13). Additionally, CspA binds C7 and C9 and blocks C9 polymerization (11). This prevents the formation of membrane attack complexes and evades MAC-mediated bacteria lysis.
B. burgdorferi is able to disseminate from the site of infection into other tissues via the blood or travelling via lymphatics. It is in these secondary lymphoid tissues that naive T cells encounter antigens for their first time and signals are sent for B cell proliferation (3). However, it has been studied that B. burgdorferi may have developed mechanisms that allows it to plays role in interfering with the initiation of the adaptive immune induction (3). Specifically, B. burgdorferi may be able to infiltrate B cells and prevent them from recruitment and proliferation so that the pathogen is able to persist for a longer period of time in these secondary sites it travels to (3).
B. burgdorferi escapes the host adaptive immune response through antigenic variation. Antigenic variation is when the pathogen surface molecules are altered in order to avoid recognition by the host immune system. B. burgdorferi achieves this through random segmental recombination of genes at the vls locus. The vls locus contains the vlsE gene as well as many vls silent cassettes that are variations of vlsE (3) which are randomly utilized during gene recombination events. This is also seen with OspC, which is a bacterial lipoprotein important for initial colonization. However, it is downregulated after infection is established to avoid antibody targeting. a T-dependent antigen that is essential for the initial colonization of mammalian hosts. Shortly after infection, this lipoprotein is no longer required, and if not down-regulated triggers a strong and effective antibody response (3,15). These events allow the evasion of humoral immune response and persistence of B. burgdorferi in hosts.
B cell functionality is also not optimal in B. burgdorferi infections. While there is a robust amount of antibodies made during immune responses, the quality of the antibody response may not be the best as demonstrated by the long-term survival of the bacteria in the host. IgM is the first antibody isotype produced and functions in limiting bacteremia in blood and activating the classical complement pathway. Usually, B cells undergo class-switching to produce IgG that is more efficient in clearing infections (16). However, during B. burgdorferi infection, serum IgM levels remain high throughout infection and IgG levels are lower than expected. This is due to the lack of sustained germinal center formations, where class-switching takes place. Defect T-dependent germinal centers are formed due to Tfh level decline as well as follicular dendritic cell mislocalization (4, 17). The mechanism of bacterial inhibition of germinal center formation is unknown. It is thought to be linked to B. burgdorferi interference with the complement system. Complement components are involved in continuous antigen presentation, which is essential for germinal center activities. B. burgdorferi inhibits C3 and C4 formation through inhibiting C3 proconvertase and C1 complex activities, respectively (11,12). C3 and C4 are unable to be deposited on FDCs, which interferes with FDC antigen presentation to B cells, which may lead to the premature collapse of germinal centers before it can function properly (18). The suboptimal T-dependent B cell class-switching is exploited by B. burgdorferi for long-term survival in hosts.
References:
- Hagen A. The bulls-eye rash of Lyme disease: Investigating the cutaneous host-pathogen dynamics of erythema migrans. [Internet]. American Society for Microbiology. 2018 [cited 11 February 2022]. Available from: https://asm.org/Articles/2018/April/going-skin-deep-investigating-the-cutaneous-host-p
- Coburn J, Garcia B, Hu L, Jewett M, Kraiczy P, Norris S et al. Lyme Disease Pathogenesis. Current Issues in Molecular Biology. 2022;42:473-518.
- Tracy K, Baumgarth N. Borrelia burgdorferi Manipulates Innate and Adaptive Immunity to Establish Persistence in Rodent Reservoir Hosts. Frontiers in Immunology. 2017;8(1664-3224).
- Anderson C, Brissette CA. The Brilliance of Borrelia: Mechanisms of Host Immune Evasion by Lyme Disease-Causing Spirochetes. Pathogens. 2021 Mar;10(3):281.
- Nuttall PA. Tick saliva and its role in pathogen transmission. Wiener klinische Wochenschrift. 2019 May 6:1-2.; Schwan TG, Piesman J, Golde WT, Dolan MC, Rosa PA. Induction of an outer surface protein on Borrelia burgdorferi during tick feeding. Proceedings of the national academy of sciences. 1995 Mar 28;92(7):2909-13.
- Schuijt TJ, Hovius JW, van Burgel ND, Ramamoorthi N, Fikrig E, van Dam AP. The tick salivary protein Salp15 inhibits the killing of serum-sensitive Borrelia burgdorferi sensu lato isolates. Infection and immunity. 2008 Jul;76(7):2888-94.
- Troxell B, Xu H, Yang XF. Borrelia burgdorferi, a pathogen that lacks iron, encodes manganese-dependent superoxide dismutase essential for resistance to streptonigrin. Journal of Biological Chemistry. 2012 Jun 1;287(23):19284-93.
- Chung Y, Zhang N, Wooten RM. Borrelia burgdorferi elicited-IL-10 suppresses the production of inflammatory mediators, phagocytosis, and expression of co-stimulatory receptors by murine macrophages and/or dendritic cells. PLoS One. 2013 Dec 19;8(12):e84980.
- Showman A, Aranjuez G, Adams P, Jewett M. Gene bb0318 Is Critical for the Oxidative Stress Response and Infectivity of Borrelia burgdorferi. Infection and Immunity. 2016;84(11):3141-3151.
- Ramsey M, Hyde J, Medina-Perez D, Lin T, Gao L, Lundt M et al. A high-throughput genetic screen identifies previously uncharacterized Borrelia burgdorferi genes important for resistance against reactive oxygen and nitrogen species. PLOS Pathogens. 2017;13(2):e1006225.
- Skare J, Garcia B. Complement Evasion by Lyme Disease Spirochetes. Trends in Microbiology. 2020;28(11):889-899.
- Caine J, Lin Y, Kessler J, Sato H, Leong J, Coburn J. Borrelia burgdorferi outer surface protein C (OspC) binds complement component C4b and confers bloodstream survival. Cellular Microbiology. 2017;19(12):e12786.
- Kenedy M, Vuppala S, Siegel C, Kraiczy P, Akins D. CspA-Mediated Binding of Human Factor H Inhibits Complement Deposition and Confers Serum Resistance in Borrelia burgdorferi. Infection and Immunity. 2009;77(7):2773-2782.
- Norris S. vls Antigenic Variation Systems of Lyme Disease Borrelia: Eluding Host Immunity through both Random, Segmental Gene Conversion and Framework Heterogeneity. Microbiology Spectrum. 2014;2(6).
- Tilly K, Krum J, Bestor A, Jewett M, Grimm D, Bueschel D et al. Borrelia burgdorferi OspC Protein Required Exclusively in a Crucial Early Stage of Mammalian Infection. Infection and Immunity. 2006;74(6):3554-3564.
- Collins A. IgG subclass co‐expression brings harmony to the quartet model of murine IgG function. Immunology & Cell Biology. 2016;94(10):949-954.
- Elsner R, Hastey C, Baumgarth N. CD4+T Cells Promote Antibody Production but Not Sustained Affinity Maturation during Borrelia burgdorferi Infection. Infection and Immunity. 2014;83(1):48-56.
- Donius L, Weis J, Weis J. Murine complement receptor 1 is required for germinal center B cell maintenance but not initiation. Immunobiology. 2014;219(6):440-449.
iv) Outcome: is the bacteria completely removed, does the patient recover fully and is there immunity to future infections from this particular bacteria?
In most cases of prompt diagnosis and taking antibiotics, such as doxycycline or hygromycin A, during early stages, B. burgdorferi can be completely removed and the patient will be completely cured in 2-4 weeks of taking oral antibiotics (1).
Approximately 15 percent of patients treated with the recommended antibiotics will have disease symptoms that persist after they complete treatment, including joint or muscle aches, cognitive dysfunction, and fatigue (2). This condition is called post-Lyme disease syndrome (PLDS), the pathogenesis of which has been up for debate for many years. Patients may feel the lingering effects of this condition for over 6 months after they finish initial treatment (3). Some researchers postulate that PLDS occurs because hosts may harbor hidden reservoirs of B. burgdorferi after their treatment, but this has neither been confirmed nor denied by evidence (3). Other theories regarding PTLDS suggest that the initial infection of B. burgdorferi introduces the triggering of auto immune responses (3). There is currently no known treatment for PTLDS; certain studies involved the prolonged usage of antibiotics, but their experimental groups showed no significant improvement compared to individuals on placebo (3). In addition, prolonged or incorrect usage of antibiotics can lead to further complications, cause serious damage to various internal organs such as the kidney, and in some cases death (3). Specifically, doxycycline antibiotics can disrupt gut microbiome, therefore weakening the host’s natural microbiota flora following usage (4).
In most cases, immunity will be developed only for the specific strain of B. burgdorferi that the individual was infected with. Antigenic variations of B. burgdorferi allow them to evade the antigen-specific immune responses previously developed from infection. Therefore, infection with these pathogen alternatives will not elicit responses from existing antibodies or immune cells (5). For individuals who experienced early and late manifestations of B. burgdorferi infection, the host immune response is rendered more developed to the surface proteins of B. burgdorferi such as OspA and other antigens (6). However, this immunity is not shown to be guaranteed as reinfection of the same B. burgdorferi strain is still possible (7). One of the most common reasons for reinfection is that the individuals are still in contact with areas that are prone to tick exposure on a day to day basis. To avoid this, a number of preventative measures can be taken to limit reinfection such as ensuring full skin coverage in tick prone areas and to use repellant. The symptoms of reinfection mirror that of initial infection. The most characteristic sign would be the appearance of Erythema migrans with presence of punctum (that shows where the tick had originally attached). Other symptoms following reinfection resemble that of the flu, exhibiting same levels of intensity as initial infection (8). It is only until a third episode of infection that the symptoms reduce in intensity (8). The susceptibility of reinfection suggests that initial infection may not elicit long-term protective immunity (9). Potential factors that may explain this phenomenon include the inability of the host to form sustained germinal centers where class switching and memory B cell formation occurs. The lower levels of IgG and memory B cell generation can then reflect the unsuccessful attempts of the host immune system at resolving subsequent infections (9).
In the cases where treatment or diagnosis of B. burgdorferi are delayed, more long term conditions may develop. For individuals who did not receive any treatment (or late treatment), they are at a predisposed position of risk for relapse (distinctly different from reinfection). Relapse would be characterized as prolonged infection of B. burgdorferi and usually occurs within a year of the initial infection (10). The difference between reinfection and relapse can be characterized by seasonality, presence of punctum and timeline of sickness. Relapse does not depend on the usual tick season (spring or summer), and no punctum is observed (10).
References
- CDC. Lyme disease frequently asked questions (FAQ) [Internet]. Centers for Disease Control and Prevention. 2021 [cited 2022 Feb 13]. Available from: https://www.cdc.gov/lyme/faq/index.html
- Melia MT, Auwaerter PG. Time for a different approach to Lyme disease and long-term symptoms. N Engl J Med. 2016 Mar 31;374(13):1277-8.
- CDC Editors. Post-Treatment Lyme Disease Syndrome [Internet]. Centers for Disease Control and Prevention. 2022 [cited 11 February 2022]. Available from: https://www.cdc.gov/lyme/postlds/index.html
- A targeted antibiotic for treating lyme disease [Internet]. National Institutes of Health. U.S. Department of Health and Human Services; 2021 [cited 2022Feb18]. Available from:https://www.nih.gov/news-events/nih-research-matters/targeted-antibiotic-treating-lyme-disease
- Frank SA. Chapter 3, Benefits of Antigenic Variation. In: Immunology and Evolution of Infectious Disease. Princeton, NJ: Princeton University Press; 2002.
- Heikkila T, Seppala I, Saxen H, Panelius J, Yrjanainen H, Lahdenne P. Species-specific serodiagnosis of Lyme arthritis and neuroborreliosis due to Borrelia burgdorferi sensu
- Elsner R, Barthold S, Baumgarth N. The immune system cannot generate immunological memory during infection with the Lyme disease agent B. burgdorferi. Cytokine. 2013;63(3):261.
- Krause PJ, Foley DT, Burke GS, et al. Reinfection and relapse in early Lyme disease, Am J Trop Med Hyg, 2006, vol. 75 (pg. 1090-4)
- Khatchikian C, Nadelman R, Nowakowski J, Schwartz I, Wormser G, Brisson D. Evidence for Strain-Specific Immunity in Patients Treated for Early Lyme Disease. Infection and Immunity. 2014;82(4):1408-1413.
- Nadelman RB, Wormser GP. Reinfection in patients with lyme disease [Internet]. OUP Academic. Oxford University Press; 2007 [cited 2022Feb18]. Available from: https://academic.oup.com/cid/article/45/8/1032/344607