Course:CONS200/2021/Radioactivity, viruses and greenhouse gases: The impacts of melting permafrost in the Arctic
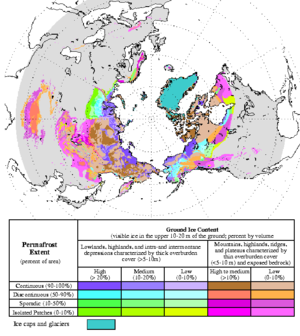
Permafrost refers to ground that continuously remains below 0 °C for two or more years, located on land or under the ocean. Permafrost includes the first layer that is on the ground, and from an inch to several miles deep under the land surface. Most permafrosts are located in the Northern Hemisphere, mainly in the Arctic. Around 15% of the Northern Hemisphere or 11% of the global surface is underlain by permafrost, including substantial areas of Alaska, Greenland, Canada and Siberia [2].
Permafrost contains large amounts of biomass and decomposed biomass that has been stored as methane and carbon dioxide in the permafrost, making the tundra soil a carbon sink, like a giant freezer. As global warming heats the ecosystem and causes soil thawing, the permafrost carbon cycle accelerates and releases much of these soil-contained greenhouse gases into the atmosphere, creating a feedback cycle that increases climate change[3]. As the soil melts, it also releases ancient viruses and radioactive gases and wastes into the atmosphere, causing serious concerns.
Nature of the issue
Thawing
In the northern circumpolar region, permafrost contains 1700 billion tons of organic material equaling almost half of all organic material in all soils[4]. This pool was built up over thousands of years and is only slowly degraded under the cold conditions in the Arctic. The amount of carbon sequestered in permafrost is four times the carbon that has been released to the atmosphere due to human activities in modern times[5]. During thaw, the ice content of the soil melts and, as the water drains or evaporates, causes the soil structure to weaken and sometimes become viscous until it regains strength with decreasing moisture content. Thawing can also influence the rate of change of soil gases with the atmosphere[6]. Arctic permafrost has been diminishing for many centuries. The consequence is thawing soil, which may be weaker, and release of methane, which contributes to an increased rate of global warming as part of a feedback loop caused by microbial decomposition[7]. Wetlands drying out from drainage or evaporation compromises the ability of plants and animals to survive. When permafrost continues to diminish, many climate change scenarios will be amplified. In areas where permafrost is high, the infrastructure surrounded may be damaged severely by the thawing of permafrost[8]. It is estimated that 16 to 24% of Alaska's permafrost will degrade by 2100[9].
Predicted rate of change in the Arctic
According to IPCC Fifth Assessment Report there is high confidence that permafrost temperatures have increased in most regions since the early 1980s. Observed warming was up to 3 °C in parts of Northern Alaska (early 1980s to mid-2000s) and up to 2 °C in parts of the Russian European North (1971–2010)[10]. In Yukon, the zone of continuous permafrost might have moved 100 kilometres (62 mi) poleward since 1899, but accurate records only go back 30 years. It is thought that permafrost thawing could exacerbate global warming by releasing methane and other hydrocarbons, which are powerful greenhouse gases[11]. It also could encourage erosion because permafrost lends stability to barren Arctic slopes[12].
Arctic temperatures are expected to increase at roughly twice the global average rate[13]. The Intergovernmental Panel on Climate Change (IPCC) will in their fifth report establish scenarios for the future, where the temperature in the Arctic will rise between 1.5 and 2.5 °C by 2040 and with 2 to 7.5 °C by 2100. One estimate suggests that 110–231 billion tons of CO2 equivalents will be emitted by 2040, and 850–1400 billion tons by 2100[14]. This corresponds to an average annual emission rate of 4–8 billion tons of CO2 equivalents in the period 2011–2040 and annually 10–16 billion tons of CO2 equivalents in the period 2011–2100 as a result of thawing permafrost. For comparison, the anthropogenic emission of all greenhouse gases in 2010 is approximately 48 billion tons of CO2 equivalents[15]. Release of greenhouse gases from thawed permafrost to the atmosphere increases global warming[16].
Consequences
Radioactivity
Like viral biomass and greenhouse gases, radioactive material stored within the Earth’s permafrost layer is becoming exposed due to human activity. Radioactivity in permafrost has two sources, natural and artificial. Natural radiation comes from the decay of radioactive isotopes naturally occurring within the crust, with product isotopes like radon gas being stored within the frozen permafrost. Artificial sources of radiation are more recent developments since the 1940’s. Since the 1940’s humans have been developing and testing large scale nuclear projects from weapons programs to power plants. The result of these tests and accidents surrounding them are the causes of substantial amounts of nuclear waste buildups within the permafrost layer [17]. Radioactive plutonium and caesium are detected today in sea bottom sediments and ice sheets, and in plants and soil beneath glaciers. Moreover, The U.S. Camp Century, a nuclear-powered research center in Greenland, generated radioactive waste that was abandoned beneath the ice when the site was decommissioned in 1967. That ice is now rapidly retreating, with losses of about 268 tons (243 metric tons) per year, as the Arctic warms. It is claimed that the Arctic radiation levels could remain harmful until 2500[17]. These newly uncovered sources of radiation may spell dangerous health conditions for both the humans and wildlife in the regions with melting permafrost.
Viruses
For centuries humans have evolved and learned to survive a vast majority of diseases through resistance, from smallpox to the plague. The increase in the Earth’s temperature and thawing of ancient ice release viable viruses, bacteria, fungi, and other microorganisms which were trapped for thousands and millions of years. Such microorganisms may belong to novel microbial species, unknown genotypes of present pathogens, already eradicated pathogens, or even known pathogens that gained extremely robust characteristics due to their subjection to long-term stress. This exposure could potentially be catastrophic. Viruses and bacteria are very closely tied to other aspects of the melting permafrost, such as climate change; temperature shifts affect the rate of development, reproduction, and survival of pathogens[18]. A 2016 anthrax outbreak in Siberia from a bacteria-infected reindeer carcass caused over 70 people to be hospitalized in northern Russia, and killed a child and more than 2,300 reindeer[19]. Reconstruction of smallpox and Spanish flu genomes from ancient frozen biological samples[18] have also demonstrated the potential threat.
Greenhouse gases
Packed with many thousands of years of life, permafrost is one of earth’s great stores of global warming gases. Large quantities of methane are stored in the Arctic in natural gas deposits, in permafrost, and as submarine clathrates. Permafrost and clathrates degrade on warming, and thus, large releases of methane from these sources may arise as a result of global warming[20]. Permafrost in the Arctic alone is estimated to hold nearly twice as much carbon as exists in the atmosphere now, as well as a sizeable amount of methane, a powerful greenhouse gas that traps more than 80 times more heat on the planet than carbon does. Preliminary computer analyses suggest that permafrost could produce carbon equal to 15 percent or so of today's emissions from human activities.According to one study, as much as 92 billion tons of carbon could be emitted between now and 2100[21]. The Arctic is warming more than two times faster than the global average[19]. Permafrost thaw contributes to a positive feedback loop that further accelerates the warming of Earth, releasing methane, which is a more powerful greenhouse gas than carbon, directly into the atmosphere, and contributing to the spread of devastating Arctic wildfires. Though its lifespan in the atmosphere is much shorter than carbon dioxide, methane’s impact on climate change has been found to be 25 times greater over a 100-year period[22]. While the Arctic was previously considered a carbon sink, new research shows that the region is emitting more carbon than it is absorbing, largely due to permafrost thaw. It is estimated that the world’s permafrost contains up to 1,700 billion tonnes of carbon, which is almost double the amount of carbon in the Earth’s atmosphere, and four times more than what has already been emitted by humans since the Industrial Revolution[23]. If all permafrost were degraded to the point of decomposing, the disastrous effects would be felt all over the world.
Future remedial actions
The UN Sustainable Development Goals
Given the influence of global processes on the Arctic and permafrost, it is critical that we make the connection between global work on sustainable development and Arctic work. Finland, the current chair of the Arctic Council, made it a priority to look to the United Nations Sustainable Development Goals (SDGs)—adopted by the nation-states of the world—to serve as the council’s guiding framework. It is crucial that the council continue this work because it will inform the UN’s post-2030 development agenda[24]. Unfortunately, global sustainable development frameworks still do not reflect the Arctic’s priorities, although there are goals that indirectly help the Arctic by taking climate actions such as Goal 13. Since the Arctic and its unique features are critically important to the health of our planet, further actions should be taken to include the Arctic in the SDGs. Sustainable development in the Arctic is going to be very difficult if the rest of the world does not understand how its actions affect the region, and cannot or will not acknowledge its responsibility. This work should highlight the fact that the actions of faraway nations have dire consequences for sustainable development in the Arctic[24].
Climate mitigation
The most effective solution for the thawing permafrost is through climate mitigation. Although climate change cannot be completely stopped, it can be slowed. A consecutive and collaborative approach to reduce the human carbon footprint via climate mitigation would not only improve the condition of the rapidly melting permafrost but would also improve the general state of the earth. Mitigation strategies include retrofitting buildings to make them more energy efficient; adopting renewable energy sources like solar, wind and small hydro; helping cities develop more sustainable transport such as bus rapid transit, electric vehicles, and biofuels; and promoting more sustainable uses of land and forests[25].
Climate adaptation
Where climate mitigation methods like lowering the global human carbon footprint work to stop changes in global climate before they occur, climate adaptation works to cope with the effects of climate change after they occur. Should all efforts to stop melting fail, humanity would likely still live on having to cope with the effects of Arctic ice melting. Adaptations to melting Arctic ice and subsequent mass emissions of greenhouse and radioactive gases primarily take the form of capturing released gases or preparing populations for increased radiation levels.
The mass emission of greenhouse gases due to melting Arctic ice creates a positive feedback loop of ice melting. This feedback loop through the emission of greenhouse gases can be slowed through the introduction of carbon capture plants and sustainable land use. Carbon capture plants work by flowing air through large machines made to scrub carbon dioxide and other gases from air[26]. These carbon capture plants could be set up around the Arctic relying on locally available renewable energy sources to indefinitely remove greenhouse gases released from the Arctic ice. Other greenhouse gases like methane may be captured as well which (if available in high enough volumes) could be exported to nations for energy usage.
Radioactive isotopes currently stored within Arctic ice present further issues when considering the melting permafrost. Where greenhouse gases may be partially removed through carbon sequestration methods like carbon capture, radioactive emissions aren’t as easily dealt with. The adaptations required to deal with radioactivity primarily take the form of having to deal with the harm done by radiation like increased rates of cancer. Luckily the previously mentioned carbon capture methods do slow the rates of ice melting meaning the buildup of radiation is slowed for some time allowing partial decay of isotopes to potentially less dangerous ones.
Besides indirect removal through delaying the release of radioactive isotopes, radioactive material can be adapted to through physical removal. Radioactive material from power plants, disasters and weapons tests that has spread to the Arctic permafrost over the years can be partially fixed through the removal of topsoil. Seen after nuclear disasters, medium half-life radioactive isotopes like Caesium-137 have been found in rain after the Chernobyl and Fukushima disasters[27]. Due to the ever moving air masses of our atmosphere, similar radioactive isotopes can be moved to polar regions and have been trapped within the permafrost. Once these contaminated permafrosts melt the water runoff will travel downstream and concentrate in larger bodies of water[28]. These radioactive bodies of water present large issues as the water could potentially cause disease and cancer for those living around them.
In these cases of concentration of radioactive runoff, top soil removal can be used to reduce risks of radiation. Used in places like Chernobyl Ukraine, Fukushima Japan along with countless other places topsoil removal has been used to reduce radioactivity to safe levels. While not at the same scale as in these disaster areas, the practice of topsoil removal could be used to skim off depositions of radioactive isotopes released from permafrost melt.
Conclusion
The thawing of the permafrost has a significant impact on not only the ecosystem, but also the carbon cycle on a global scale. It reminds us of just how grim the climate crisis is. As the permafrost thaws it releases copious amounts of greenhouse gasses, radioactivity, viruses, and waste all of which pose a serious threat to the health and wellbeing of all the biodiversity present on this earth. The need to implement solutions is more crucial than ever. To prevent and mitigate the further harmful effects of the thawing permafrost, actions must be taken immediately; the most effective action being climate mitigation, through a collective global approach climate change can be slowed dramatically, in turn reducing the rate at which the permafrost melts. Climate adaptation is also a very effective solution; as stated previously climate change cannot be stopped it can only be slowed, adapting to the climate is essential in order to safeguard the health of humans and all biodiversity. The melting permafrost is a simple example of how climate change can severely disrupt our ecosystem and the quality of life of everything that lives within it.
References
- ↑ Brown, J., O.J. Ferrians, Jr., J.A. Heginbottom, and E.S. Melnikov. 1998, revised February 2001. Circum-arctic map of permafrost and ground ice conditions. Boulder, CO: National Snow and Ice Data Center/World Data Center for Glaciology - http://nsidc.org/data/docs/fgdc/ggd318_map_circumarctic/index.html
- ↑ Obu, J. (2021). How much of the Earth's surface is underlain by permafrost? Journal of Geophysical Research: Earth Surface, 126(5). https://doi.org/10.1029/2021jf006123e
- ↑ Denchak, Melissa (2018). "Permafrost: Everything You Need to Know". NRDC.
- ↑ Tarnocai; et al. (2009). "Soil organic carbon pools in the northern circumpolar permafrost region". Global Biogeochemical Cycles. 23 (2): GB2023. doi:10.1029/2008gb003327.
- ↑ Schuur; et al. (2011). "High risk of permafrost thaw". Nature. 480 (7375): 32–33. Bibcode:2011Natur.480...32S. doi:10.1038/480032a.
- ↑ Kim, D; Vargas, R; Bond-Lamberty, B; Turetsky, M (2012). "Effects of soil rewetting and thawing on soil gas fluxes: a review of current literature and suggestions for future research". Biogeosciences. 9: 2459-2483. doi:10.5194/bg-9-2459-2012.
- ↑ Koven, Charles D.; Riley, William J.; Stern, Alex (2012-10-01). "Analysis of Permafrost Thermal Dynamics and Response to Climate Change in the CMIP5 Earth System Models". Journal of Climate. 26 (6): 1877–1900. doi:10.1175/JCLI-D-12-00228.1.
- ↑ Nelson, F. E.; Anisimov, O. A.; Shiklomanov, N. I. (2002-07-01). "Climate Change and Hazard Zonation in the Circum-Arctic Permafrost Regions". Natural Hazards. 26 (3): 203–225. doi:10.1023/A:1015612918401.
- ↑ "THAWING PERMAFROST". WWF. 2019.
- ↑ Alexander; et al. (2013). "Working Group I Contribution to the IPCC Fifth Assessment Report Climate Change 2013 – Summary for Policymakers". TemplateLAB. Explicit use of et al. in:
|last=
(help) - ↑ Reuters (2019-06-18). "Scientists shocked by Arctic permafrost thawing 70 years sooner than predicted". The Guardian. ISSN 0261-3077.
- ↑ Turetsky, Merritt R. (2019-04-30). "Permafrost collapse is accelerating carbon release". Nature. 569 (7754): 32–34. Bibcode:2019Natur.569...32T. doi:10.1038/d41586-019-01313-4.
- ↑ Scott, Michon (2021). "2020 Arctic air temperatures continue a long-term warming streak". National Oceanic and Atmospheric Administration.
- ↑ Schuur; et al. (2011). "High risk of permafrost thaw". Nature. 480 (7375): 32–33. Bibcode:2011Natur.480...32S. doi:10.1038/480032a. PMID 22129707. S2CID 4412175.
- ↑ UNEP 2011. Bridging the Emissions Gap. A UNEP Synthesis Report. 56 p. UNEP, Nairobi, Kenya
- ↑ Comyn-Platt, Edward (2018). "Carbon budgets for 1.5 and 2 °C targets lowered by natural wetland and permafrost feedbacks". Nature Geoscience. 11 (8): 568–573. doi:10.1038/s41561-018-0174-9.
- ↑ 17.0 17.1 Miner, K. R., D’Andrilli, J., Mackelprang, R., Edwards, A., Malaska, M. J., Waldrop, M. P. & Miller, C. E. (2021). Emergent biogeochemical risks from Arctic permafrost degradation. Nature Climate Change, 11(10), 809–819. https://doi.org/10.1038/s41558-021-01162-y
- ↑ 18.0 18.1 El-Sayed, Amr (2020). "Future threat from the past". Nature Public Health Emergency Collection – via NCBI.
- ↑ 19.0 19.1 Bykova, Alina (2020). "Permafrost Thaw in a Warming World: The Arctic Institute's Permafrost Series Fall-Winter 2020". The Arctic Institute.
- ↑ Pfeiffer, Eva-Maria; Grigoriev, Mikhail N.; Liebner, Susanne; Beer, Christian; Knoblauch, Christian (April 2018). "Methane production as key to the greenhouse gas budget of thawing permafrost". Nature Climate Change. 8 (4): 309–312. doi:10.1038/s41558-018-0095-z.
- ↑ Schuur, E. A. G (April 2015). "Climate change and the permafrost carbon feedback". Nature. 520: 171–179 – via ResearchGate.
- ↑ Weston, Pheobe (2019). "Thawing permafrost turns Arctic from carbon sink into carbon emitter, study finds". Independent.
- ↑ Kopansky, Dianna (2019). "Thawing Arctic peatlands risk unlocking huge amounts of carbon". UN Environment Programme.
- ↑ 24.0 24.1 Koivurova, Timo (2018). "Why the Arctic needs the UN Sustainable Development Goals". WWF.
- ↑ "Climate Change Mitigation". Global Environment Facility. 2021.
- ↑ Boot-Handford, M. E., Abanades, J. C., Anthony, E. J., Blunt, M. J., Brandani, S., Mac Dowell, N., ... & Fennell, P. S. (2014). Carbon capture and storage update. Energy & Environmental Science, 7(1), 130-189.
- ↑ Hinrichsen, Y., Finck, R., Martinsson, J., & Rääf, C. (2021). Maximizing avertable doses with a minimum amount of waste for remediation of land areas around typical single family houses after radioactive fallout based on Monte Carlo simulations. Scientific reports, 11(1), 1-9.
- ↑ Song, C., Wang, G., Liu, G., Mao, T., Sun, X., & Chen, X. (2017). Stable isotope variations of precipitation and streamflow reveal the young water fraction of a permafrost watershed. Hydrological Processes, 31(4), 935-947.
This conservation resource was created by Course:CONS200. It is shared under a CC-BY 4.0 International License. |