PHYS341/2024/Project23
Guitar String Vibrations
Introduction: The Guitar
The guitar has gained global recognition due to its tunefulness and adaptability, which allows for a wide range of ways of playing it and different guitar-making traditions[1]. However, what fascinates me about guitar strings is how manipulating them affects sound production. When plucked, the strings vibrate, transferring energy to the guitar's body and the surrounding air. This vibration is then amplified within the guitar’s cavity, enhancing the sound we hear.[2] Nevertheless, we will explore how altering the length, thickness, and tension of a string can further impact sound production, demonstrating the intricate relationship between a string’s physical properties and the resulting acoustic qualities.
Historical Background
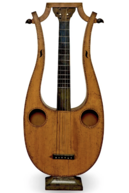
Originating as a simple stringed instrument, the guitar has evolved significantly, enhancing playability and sound, culminating in the electric guitar[1]. Figure 2 illustrates a historical lyre-shaped guitar from the 19th century.
The guitar originated in ancient cultures in medieval Europe and was transformed tremendously.
- By the sixteenth century, it had begun evolving into something like the classical guitars we know today, with electric guitars emerging in the twentieth century as a significant improvement in sound amplification.
- In the nineteenth century, Antonio Torres's evolution of this guitar from Renaissance lute-like instruments to classical guitars laid the foundation for present-day acoustic and electric guitars.
- Presently, every new approach develops sound production, keeping musical expression pace with progress in physics and material science.[1]
Acoustic Guitar Construction
Both acoustic and electric guitars usually feature steel strings, while classical guitars use nylon strings. The bottom three strings on classical guitars, which produce high treble tones, are made of a single, clear nylon filament, while the top three, producing lower bass tones, consist of nylon strands wrapped in silver-plated copper wire[3][4][5].
A typical Western acoustic guitar includes a body, neck, and headstock, with six strings spanning from bridge to tuning pegs. The measurements of the acoustic steel string guitar used are:
- Body Length: 50.2 cm
- Neck Length: 36.3 cm
- Total String Length: 66.1 cm
Sound Waves and Spectral Analysis
The production of sound from a guitar starts from the vibration of its strings. When a string is plucked or strummed, it initiates motion, generating standing waves crucial for sound production. Playing involves manipulating the strings with one hand while altering their vibrating length with the other by pressing down on the fretboard, thus varying pitches[3].
String Vibrations and Waveforms - Nylon vs. Steel String
Sound waves are visually represented as waveforms, showcasing the oscillations that form the backbone of guitar sound. Guitars can have steel or nylon strings, which can produce the same frequencies, but there are differences in the waveforms and sound quality.
Waveforms:
- Nylon Strings: The waveform produced by nylon strings show a smoother, more rounded shape with less sharp peaks, indicating softer motion with less sustain.
- Steel Strings: The waveforms produced by steel strings display sharper, more pronounced peaks, reflecting a metallic tone with longer sustain.
Amplitude and Decay:
- Nylon Strings: Nylon strings might show a faster decay in amplitude, meaning the note fades out more quickly.
- Steel Strings: Steel strings, which are more rigid, exhibit a slower decay, so the note plays longer.
Spectral Analysis - Steel String Only
In the spectral analysis of the low E string on a steel-string guitar shown in Figure 5a, the fundamental frequency is approximately 82 Hz, corresponding to the E2 note. This primary pitch is accompanied by higher frequencies known as overtones or harmonics, visible as additional peaks in the spectral graph. This illustrates that any sound can break down into pure tones with varying amplitudes and frequencies[6].
The fundamental frequency and its harmonics merge through the principle of superposition to produce a complex sound composed of various frequencies.
The waveform representation of the low E string shown in Figure 5b illustrates the principle of superposition in action. This principle explains how multiple waves can travel through the same medium simultaneously without disturbing each other, and when the waves meet, they combine to form a new wave pattern (which is the sum of the displacement of the individual pure tone waves)[6].
Factors Influencing Sound Production for Acoustic Guitar with Steel Strings
Background
How string length, thickness, and tension affect the fundamental frequency can be described by the equations and , where:
- f is the frequency
- v is the wave speed
- λ is the wavelength
- L is the length of the string
- T is the tension force
- μ (mu) is the mass per length
combines to give .[7]
Length
For a stretched string, the fundamental frequency is given by . Additionally, for harmonic frequencies, wavelengths are twice the length of the string and anything you can get by dividing that by a whole number as noted by , where fn is the frequency of the n-th harmonic and n is a positive integer (1, 2, 3, ...)[7][8].
Effects of string length on sound frequency were explored using the guitar's sixth string, the low E. As indicated in Figure 6, three notes—E2, A2, and C3—were played, representing a gradual string shortening as we moved up the fretboard (see Figure 7). According to Figure 6, the frequency increased as the string's effective length decreased as the string was shortened by playing the three notes, aligning with the idea that frequency is inversely proportional to string length.
Thickness
Each guitar string was tuned to standard pitches E-A-D-G-B-E, and their open notes were recorded by plucking the corresponding string shown in Figure 9[4].
Spectral Analysis shown in Figure 8 revealed that thinner strings produced higher frequencies, which can be partially attributed to their lower mass. The physics principle here involves the inverse relationship between mass per unit length square root and frequency, as given by where a smaller μ for thinner strings results in a higher f. This is a way of altering wave speed in order to impact the frequency.[7]
Tension
The tension experiment was conducted on the tuned sixth string (Figure 10a). The tension on the string was increased (Figure 10b) after recording the open E note, raising the pitch. Increasing the tension resulted in a higher frequency played which aligns with . This showcases the direct proportionality between tension and frequency, a fundamental concept in the physics of vibrating strings.[7][9]
Conclusion
Based on this exploration and , for a string fixed on both ends[7]:
- The fundamental frequency is inversely proportional to twice the length (L) of the string (since the wavelength is twice the length of the fundamental standing wave).
- The frequency is directly proportional to the square root of the tension (T).
- The frequency is inversely proportional to the mass per length (μ) square root.
Real World Applications
If nylon and steel strings have different material properties such as a lower mass per unit length, how do they produce the same frequency when an open string is plucked? This is accounted for when a guitar is tuned and done by adjusting the tension of each string until it reaches the correct pitch. So, despite different material properties, nylon and steel strings can produce the same pitch (E2 in the case of the low E string).
References
- ↑ 1.0 1.1 1.2 1.3 Encyclopædia Britannica (April 8, 2024). "Guitar".
- ↑ Van Raamsdonk, Mark (April 25, 2021). "Physics of Music 48: Stringed instruments: the importance of body and cavity vibrations". Youtube.
- ↑ 3.0 3.1 "Classical guitars are great for this - Musical Instrument Guide". Yamaha Corporation. April 8, 2024.
- ↑ 4.0 4.1 "Six strings, each with a higher pitch - Musical Instrument Guide". Yamaha Corporation. April 8, 2024.
- ↑ Radicioni, D.P.; Lombardo, V (October 2007). "A Constraint-based Approach for Annotating Music Scores with Gestural Information". Constraints (Dordrecht).
- ↑ 6.0 6.1 Van Raamsdonk, Mark (Apr 25, 2021). "Physics of Music 32: The principle of superposition, and decomposing a musical note into pure tones". Youtube.
- ↑ 7.0 7.1 7.2 7.3 7.4 Van Raamsdonk, Mark (April 25, 2021). "Physics of Music 38: predicting wave speed and frequencies on stretched strings". Youtube.
- ↑ Lopresto, Michael C. (November 2006). "Experimenting with Guitar Strings". The Physics Teacher. 44.
- ↑ Van Raamsdonk, Mark (April 25, 2021). "Physics of Music 21: Period and amplitude review and sample question". Youtube.