Injury Biomechanics in School Bus Restraint Systems
From UBC Wiki, the student-made encyclopedia.
Overview of School Bus Safety
School buses are one of the safest vehicles on the road, according to the National Highway Traffic Safety Administration (NHTSA).[1] Their yellow colour makes them highly visible.[1] They have several safety and protection features such as heavier weight and high crush standards so that bus passengers experience less crash force than other vehicles.[1] Nonetheless, “bus rollover crashes [still] [account] for 58 percent of the total bus occupant fatalities and 65 percent of the passenger fatalities.”[2] Compartmentalization is a passive safety system decided by NHTSA as the best way to protect passengers from crashes; large school buses place strong seats with energy-absorbing seat backs close together on the inside.[1] On the other hand, small school buses have lap or lap/shoulder belts.[1] Figure 1 shows a blueprint of a typical school bus seat.

In Canada, school buses require child car seats, booster seat anchors, electronic stability control systems (i.e. technology in vehicles that automatically activates the brakes to help steer and prevent losing control of the vehicle), seat belt installation and improved compartmentalization.[4][5] The type of restraint system depends on the passenger (a child under 6 would need a car or booster seat) and the regional requirements (some states in the USA require seat belts and others use compartmentalization).[6] Despite all these restraint systems, there is still a potential for severe fatal crashes. After analyzing test data, NHTSA found that in severe frontal crashes, the risk of serious neck injuries and possible abdominal injuries in children is increased when lap/shoulder belts are misused.[7] Moreover, rollovers are the most common cause of fatal crashes in school buses.[8] Based on this data, this paper aims to determine which safety restraint system provides the best protection for children under 12 (referring to the ATDs mentioned later in this paper) during school bus accidents.
To understand the biomechanics of these school bus injuries, various methods can be used to test the physiological responses and tolerances to severe crashes. Multi-body models, finite element analysis (FEA), rigid body dynamics, and sled tests with anthropomorphic test devices (ATD) can be used for analysis and validation. Likewise, physical evidence at crash sites and deformations of school buses (and other vehicles involved) can be examined to determine the dynamics of the crash; a comparison of the collected evidence with the injuries sustained by the young passengers can conclude the mechanism of the injury.[8] Furthermore, computational models and simulations can be validated via ATD responses from experiments.
Representative Work
Reports of fatal school bus crashes illustrated the most common injuries sustained by children to involve the head, neck, and shoulder.[8] For reference, data for head, neck, and chest injury criteria, via a dummy representing a 6-year-old child, from an NHTSA presentation are reviewed.[9] The Head Injury Criterion (HIC) was found to be approximately 0.48, 0.47, 0.12, 0.37, and 0.11 in a school bus crash with compartmentalization, lap belt, lap/shoulder belt, misused lap/shoulder belt, and misused lap/shoulder belt, respectively.[9] The neck injury criteria (Nij) numbers were 1.1, 1.4, 0.6, 0.9, and 0.6 for compartmentalization, lap belt, lap/shoulder belt, misused lap/shoulder belt, and another misused lap/shoulder belt, respectively.[9] Lastly, the chest injury based on acceleration was 0.54, 0.59, and 0.39 for compartmentalization, lap belt, and lap/shoulder belt.[9]
Computer Simulations
Based on prior research, two types of simulations are prevalent in current bus rollover tests: FEA and the rigid body approach. The rigid body approach demands less computational power, making it feasible to execute the test on a personal computer.[10] Rigid body simulation consists of rigid parts that can be connected by mechanical joints which try to simulate the overall human structure. Nevertheless, there are several drawbacks associated with using the rigid-body approach for simulations. For instance, it often yields inaccurate predictions of body deformation, as highlighted in a paper by Crandall et al., which points out "the lack of failure description at the tissue level, a relatively simple approximation of contact force, and an inability to accurately model body deformation" as notable limitations.[11] To better differentiate between lumped mass, rigid body, and FE model, Figure 2 shows all 3 types of model.
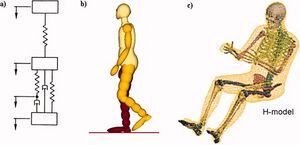
On the other hand, FEA simulations are computationally more demanding compared to the rigid body approach.[11] In the work by Saczalski et al., they introduce the Avid Softimage 3D Extreme software to enhance the realism of the occupant, vehicle, and accident scene in simulations. Simultaneously, they improve the accuracy of the simulations by comparing the results to actual incident outcomes.[12] During the data collection phase, Hybrid III and EuroSID-2re (ES-2re) with 2-point seat belts are utilized to replicate the response of a 50th percentile male during a bus rollover.[13] Researchers found that both 2-point lap belts and 3-point seatbelts significantly reduce the Abbreviated Injury Scale (AIS) score for head and neck injuries, leading to their inclusion in the simulations.[13] In other words, the 2-point seat belt is a lap belt mainly surrounding the abdominal parts, and an additional point near the neck and clavicle is included for the 3-point seat belt.
The paper by Seyedi et al. presents eight simulations, each varying due to the anthropometric differences of the ATDs. For example, Hybrid III exhibits greater stiffness in the neck compared to EuroSID 2re, resulting in ES-2re having a lower risk of neck injury in all simulations, while Hybrid III presents a relatively higher risk of neck injury.[13] Despite comparing two ATD finite element (FE) models with former years' collision data, the variability between models posed challenges for researchers in selecting the optimal simulation. Additionally, experimental data is obtained from the tilt table rollover test with HIII affixed to the seat throughout the entire test.[13]
In conclusion, the rigid body approach can provide data regarding the time, location, and critical injuries experienced by an unbelted occupant. It also suggests that belted occupants are less likely to suffer critical injuries during a bus rollover incident.[12] However, the resulting outcomes may require further verification in future studies. Conversely, two FEA simulations predict a high risk of neck and thorax injuries in case of ejection but a low risk of pelvic injury during the tilt table rollover simulation.[12] Moreover, FEA simulations offer greater accuracy when compared to the rigid body approach.[12] Therefore, the mainstream simulation in the current car crash-testing industry is FEA.[10]
ATD Testing
The desLibris of Canada Commons pointed out that school buses have a strong occupant safety record in Canada. This is mainly thanks to the numerous occupant protection elements integrated into the bus, particularly the highly efficient seat design known as compartmentalization.[14] Currently, most school buses still use compartmentalization as the main occupant protection feature, and there are no seat belts for passengers.
Chang et al. provided an overview of the historical progression of school bus safety standards highlighted concerns regarding the current limitations of compartmentalization requirements, and conducted crash tests on these buses.[15] Since school buses are mainly used for children and teenagers, passenger behaviours and seating orientation play an important role regarding safety. Their main objective was to investigate the effectiveness of compartmentalization as the sole protection feature during school bus crashes.

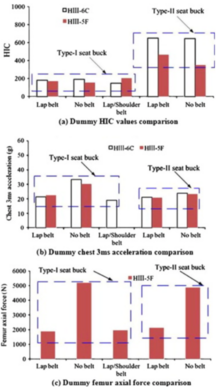
They began by performing a frontal crash test at a speed of 40 kilometres per hour on a 1998 Type C school bus, weighing over 4500 kilograms and accommodating 13 full-scale Hybrid III ATDS. These ATDs, representing a 3-year-old to a 50th percentile male, were arranged in various seating configurations—such as forward-facing, aisle-facing, and rearward-facing—to mimic the behaviours commonly seen among school children more accurately.[15]
According to their test result, the efficacy of compartmentalization is significantly constrained by several influential factors. Optimal efficacy remains contingent upon passengers assuming an upright, forward-facing posture with their backs in contact with the seat. Additionally, the ambient interior environment (for example the seats) significantly impacts passenger safety, leading to substantial variability in passenger injury outcomes across distinct crash types. For example, the seating posture of passengers, and the height of the seat are two of the common influencers on passengers' injury outcomes.[15]
Li et al. reported another series of sled tests on school bus safety in 2014. The tests were conducted according to GB24406/ECE R80 (a standard published by the Bus Standardization Sub-Committee of the National Technical Committee of Auto Standardization of China) in the Automobile Crash Laboratory of Tsinghua University.[16] They tested two different types of school bus seat belts: type-I seats are commonly used in North America and type-II seats are used under Chinese regulations. Five tests were conducted for each, as seen in Figure 3, involving the implementation of the lap belt, compartmentalization (without belt), and lap/shoulder belt restraint strategies.[16] The ATDs being used in this study are HIII-6C and HIII-5F, and the range of ATDs used is from 3-year-old to 50th male dummy.
The research team used multiple injury criteria from the Federal Motor Vehicle Safety Standard 208 (FMVSS 208) to evaluate the test results. HIC36 was used as the head injury metric;[16] the 3 ms resultant acceleration in the same safety standard was used to evaluate chest injuries.[16] While femur forces directly measured femur injuries.[16] Bar plots summarizing the test results are included in Figure 4. Comparing the results among wearing a lap belt, wearing a lap/shoulder belt and relying merely on compartmentalization, school buses would be much safer if seat belts were added as a requirement.[16]
Neck Injury Evaluation
During front-end crashes, school buses do not provide sufficient protection to the passenger’s neck.[17] Neck injuries have not been evaluated in school bus crash regulations (e.g. GB24406/ECE R80) and thus children’s neck injuries (from 3 years old to 12 years old) are commonly reported.[17] Strains and sprains accounted for the highest percentage of all injuries, followed by contusions and abrasions (28.3%) and lacerations (14.9%). Strains and sprains also occurred most frequently in the neck (37.0%).[18] A new study by Li et al. (building on their 2014 study) developed and examined multi-body seat models with different child restraint systems present in school buses: compartmentalization, lap belt, and lap/shoulder belt. These models were verified by dummy testing and neck injury metrics via sled tests.[17]
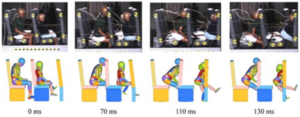
For compartmentalization, the study used MADYMO 7.5 (a software package to simulate and evaluate occupant safety systems) to build actual school bus seats including the floor, seat frame, seatback, seat cushion, and more.[17] In the seatbelt model, Li et al. combined a one-dimensional rigid lap belt model and a two-dimensional finite element lap/shoulder belt model. They tested the loading and unloading curves for both belt types. The seatback was divided into three sections because it would be impacted at different locations by different parts of the dummy (head, chest, and leg) in anterior crashes and load-deformation curves for each part would have to be defined.[17]


Li et al. used the Hybrid III 6-year-old child ATD (HIII-6C), Hybrid III 5th female ATD (HIII-5F) and P-series 3-year-old child ATD (P-3C) in their experimentation. The HIII-5F ATD represented a child of age twelve and neck injury metrics were not collected from the P-3C due to a lack of a sensor. Therefore, only data from the first two ATDs were considered; shear, axial and bending.[17] LS-DYNA, a simulation software for impacts and crashes, was used to run FE simulations to determine the contact stiffness functions between the dummies and the three-seat back sections.[17] Material properties of the foam and thick steel plate were acquired by conducting compression and tensile tests via the foam material model (MAT 57) and elastic-plastic material model (MAT 24), respectively.[17]
Using the HIII-6C and HIII-5F dummies, FE models of the head, chest, and leg were generated.[17] After restricting the sides of the seatback to make it more realistic, motion was applied to the dummy head, chest, and leg.[17] The resulting Nij can be found by deriving the neck injury metrics from FMVSS 208 and the shear force (Fx), axial force (Fz), and bending moment (My) from graphs obtained by measuring the dummies’ neck responses.[17]
To validate the models, the neck responses and kinematic trajectories of the dummies from the simulations were compared with actual experimental results.[17] The comparison was performed at the same time stamps in the simulation and sled tests. These evaluations can be seen in Figure 5(a-c), for each type of school bus restraint system. Li et al. reported high accuracy in predicting neck injury metrics in their study using these simulations and proposed an improvement to the current school bus restraint system.[17]
Limitations and Controversies
The discussion of school bus safety is one that for many years has been plagued with issues and controversy.[19] In 1995, Swartz et al. pointed out that there was a lack of school bus crash data which had led to opposition to additional school bus safety measures such as seatbelts, some even believing that seat belts would be more unsafe in rollover crashes since children may have difficulty releasing themselves.[20] Due to the lack of data, many school bus safety standards were left to school boards, who are not experts in public safety and would have to make many decisions based on finances.[21] At the time, cost-benefit analyses were conducted and found that in 1988, the cost to retrofit a bus with seat belts was $1,100 (USD) (which, ignoring any advancements in manufacturing and cost savings associated with that, would translate to $2,860 (USD) in 2023).[21] Begley et al. concluded that these additional costs would not bring meaningful benefits to student safety.[21]
Lapner et al. have also shown that compartmentalization fails to contain occupants during rollovers.[8] Furthermore, Chang et al. identified a key issue in the compartmentalization strategy is that its efficiency is reliant on student position during a crash.[15] Seat belts could help keep students in place, stopping them from moving into unideal positions in the event of a crash. Despite their obvious safety benefit, Lou et al. studied the usage of seat belts in a pilot project in the state of Alabama. It was found that students were more disciplined and behaved better when wearing seatbelts.[20] However, during their trials, they found that only 57.3% of students would use seatbelts while on these buses despite driver intervention at times.[20]
While Lou et al. investigated many reasons for the low percentage of seatbelt usage they noted that seat design may have increased the want for students to interact and socialize, something that seat belts would hinder. Safety behind seat design was also found to be lacking by Li et al. where it was found that the seat models in use contributed to neck injury.[17] An important limitation of research behind these crashes is that the children often will vary in size and these safety features have to be able to accommodate this large variation. These also can cause issues with ATD testing as it is not feasible to have an ATD for every child size, This is an advantage behind FEA testing as it can test other sizes of models much more efficiently.
Future Research
Based on previous biomechanics research, changes to the school bus design can be made to prevent these injuries. For instance, more padding can be added to the sides and around the window panels of the bus on top of compartmentalization.[17] Another energy-absorbing block can be added to the back of the seats or a revolute joint (a single-axis joint that allows rotational motion) with varying stiffness can be added between the back and the seat frame.[17][22] Similarly, a study found that children seated on the side to which the bus rolls during a rollover are susceptible to fractures.[8] This demonstrated that compartmentalization provides protection in the event of anterior or posterior collisions, but has minimal security on the sides and near windows.[8]
Today, with the advancement in biomechanics and data collection, further research has been conducted and has found that there are significant deficiencies in today's school bus safety. Many solutions have been proposed to help limit school bus collision-related injuries. Lapner et al. suggested additional padding to help with the current strategy of compartmentalization and sled tests from Li et al. seem to corroborate these findings.[8][17]
Additionally, despite the possibility of students not wearing their seat belts, it is still shown to greatly increase safety.[20] From these results, it seems clear that despite the cost, there is a large safety benefit to the addition of seatbelts and additional padding in buses. Further research should go into the seat design of bus seats, as with current data we can see the issues with compartmentalization from a biomechanics perspective.[17] However, it is important to point out that there is also a behavioural component to compartmentalization as well as the adoption of seatbelt use in students.[17][20] These reveal that further research should also be conducted on the behaviour of students and how to encourage safer actions while riding a school bus.
References
- ↑ 1.0 1.1 1.2 1.3 1.4 National Highway Traffic Safety Administration. (2023). School Bus Safety. NHTSA. https://www.nhtsa.gov/road-safety/school-bus-safety
- ↑ National Highway Traffic Safety Administration. (2021, December 29). Federal Motor Vehicle Safety Standards; Bus Rollover Structural Integrity. Federal Register :: Request Access. https://www.federalregister.gov/documents/2021/12/29/2021-27538/federal-motor-vehicle-safety-standards-bus-rollover-structural-integrity
- ↑ Code of Federal Regulations. (2023). Standard No. 222; School bus passenger seating and crash protection. 49 CFR 571.222. https://www.ecfr.gov/current/title-49/subtitle-B/chapter-V/part-571/subpart-B/section-571.222
- ↑ Transport Canada, T. C. (2020, February 27). About School Bus Safety in Canada. https://tc.canada.ca/en/road-transportation/school-bus-safety/about-school-bus-safety-canada
- ↑ Road Safety Facts. ESC: what is Electronic Stability Control and how does it work? https://roadsafetyfacts.eu/electronic-stability-control-esc-what-is-it-and-how-does-it-work/#:~:text=Electronic%20stability%20control%20(ESC)%20helps,vehicle%20in%20the%20right%20direction.
- ↑ Wonderopolis. (2014). Why Aren’t Seat Belts Required on School Buses? Wonder of the Day #1310. https://wonderopolis.org/wonder/why-arent-seat-belts-required-on-school-buses#:~:text=To%20date%2C%20only%20six%20states,require%20them%20on%20newer%20buses.
- ↑ Hinch, J., McCray, L., Prasad, A., Elias, J., Hott, C., Willke, D., & Sullivan, L. (2002, April). School Bus Safety: Crashworthiness Research. https://www.nhtsa.gov/sites/nhtsa.gov/files/sbreportfinal.pdf
- ↑ 8.0 8.1 8.2 8.3 8.4 8.5 8.6 Lapner, P. C., Nguyen, D., & Letts, M. (2003). Analysis of a school bus collision: mechanism of injury in the unrestrained child. Canadian journal of surgery. Journal canadien de chirurgie, 46(4), 269–272.
- ↑ 9.0 9.1 9.2 9.3 Hinch, J. (2002). School Bus Crashworthiness Research [PowerPoint slides]. NHTSA. https://www.nhtsa.gov/sites/nhtsa.gov/files/hinchoct2002.pdf
- ↑ 10.0 10.1 Wang, F., Yu, C., Wang, B., Li, G., Miller, K., & Wittek, A. (2020). Prediction of pedestrian brain injury due to vehicle impact using computational biomechanics models: Are head-only models sufficient?. Traffic injury prevention, 21(1), 102–107. https://doi.org/10.1080/15389588.2019.1680837
- ↑ 11.0 11.1 11.2 Crandall, J. R., Bose, D., Forman, J., Untaroiu, C. D., Arregui-Dalmases, C., Shaw, C. G., & Kerrigan, J. R. (2011). Human surrogates for injury biomechanics research. Clinical anatomy (New York, N.Y.), 24(3), 362–371. https://doi.org/10.1002/ca.21152
- ↑ 12.0 12.1 12.2 12.3 Saczalski, K., Saul, J., Harrison, T., and Lowrance, C., "Biomechanical Simulation and Animation of Vehicle Occupant Kinematics for Restrained and Unrestrained Conditions in Rollover Accidents," SAE Technical Paper 1999-01-1885, 1999, https://doi.org/10.4271/1999-01-1885.
- ↑ 13.0 13.1 13.2 13.3 Seyedi M, Jung S. Numerical assessment of occupant responses during the bus rollover test: A finite element parametric study. Proceedings of the Institution of Mechanical Engineers, Part D: Journal of Automobile Engineering. 2020;234(8):2195-2215. doi:10.1177/0954407019894425
- ↑ desLibris, Strengthening School Bus Safety in Canada, Government of Canada. Ottawa, ON, CA. Retrieved from https://canadacommons.ca/artifacts/1421237/strengthening-school-bus-safety-in-canada/2035286/ on 01 Nov 2023. CID: 20.500.12592/nwch20.
- ↑ 15.0 15.1 15.2 15.3 Chang, K., Vits, C., & Seely, B. (2015). School bus safety: Evaluating the evolution of compartmentalization and seat belt restraints. Transportation Research Record, 2513(1), 72-79. https://doi.org/10.3141/2513-09
- ↑ 16.0 16.1 16.2 16.3 16.4 16.5 16.6 16.7 Li, Z., Ge, H., Zhang, J., & Zhu, Y. (2014). The necessity of evaluating child neck injury in frontal collision of school bus for transportation safety. Safety Science, 62, 441-449. https://doi.org/10.1016/j.ssci.2013.10.00
- ↑ 17.00 17.01 17.02 17.03 17.04 17.05 17.06 17.07 17.08 17.09 17.10 17.11 17.12 17.13 17.14 17.15 17.16 17.17 17.18 17.19 17.20 17.21 Li, Z., Li, R., Zhang, H., Jiang, N., Feng, G., & Qi, X. (2020). Development of a school bus restraint system model and its application in the evaluation of designed protective strategies on child neck injury in front-end crash. International Journal of Crashworthiness, 27(3), 847–856. https://doi.org/10.1080/13588265.2020.1858644
- ↑ McGeehan, J., Annest, J. L., Vajani, M., Bull, M. J., Agran, P. E., & Smith, G. A. (2006). School bus-related injuries among children and teenagers in the united states, 2001-2003. Pediatrics (Evanston), 118(5), 1978-1984. https://doi.org/10.1542/peds.2006-1314
- ↑ Swartz, M. K., & Reilly, E. A. (1995). School Bus Safety: Issues and controversy. Journal of Pediatric Health Care, 9(3), 145–148. https://doi.org/10.1016/s0891-5245(05)80026-4
- ↑ 20.0 20.1 20.2 20.3 20.4 Lou, Y., Mehta, G., & Turner, D. S. (2011). Factors influencing students’ usage of school bus seat belts: An empirical analysis of the Alabama Pilot Project. Accident Analysis & Prevention, 43(5), 1644–1651. https://doi.org/10.1016/j.aap.2011.03.018
- ↑ 21.0 21.1 21.2 Begley, C. E., & Biddle, A. K. (1988). Cost-benefit analysis of safety belts in Texas school buses. Public health reports (Washington, D.C.: 1974), 103(5), 479–485.
- ↑ Spacetechinfo. (2023, September 6). What are Revolute Joints? – Definition, Types, Challenges, and More. Space Tech Info. https://www.spacetechinfo.com/definition/revolute-joints/